This project initiated 2012
Copyright TXu 1-795-975, TXu 1-809-958, TXu 1-823-034, TX 7-630-173 and TXu 1-852-686
“When the solar irradiance propagates between the outer magnetospheric regions and the ionosphere, dynamic processes of the magnetosphere-ionosphere-thermosphere system are affected at the lower end of their paths by the interaction of radiation with the neutral troposphere. The main target of this work is to investigate the relationship between the diurnal magnetic field variations resulting from solar activities and the variation in the troposphere temperature. Meteorological and geomagnetic data acquired from different observatories located in Egypt, Portugal and Slovakia in a long-term and daily-term scales were analyzed. The long-term results show that there is a close relationship between the diurnal Sq magnetic field variations and the tropospheric temperature. The rate of temperature increase at mid-latitude areas is higher than at high-latitude. During the period of investigation, it is found that the troposphere temperature has increased by about 0.033 °C/year at Helwan, Egypt, 0.03 °C/year at Coimbra, Portugal, and 0.028 °C/year in Hurbanovo/Stará Lesná, Slovakia. The Sq geomagnetic variations depend on the intensity of the electric currents generated by the effect of solar radiation in the ionosphere.”
SOLAR RADIATION AND GEOMAGNETIC FIELD
"Reid (1987) used in his correlation the global mean value of sea surface temperature (SST) (see Fig. 1) for the period from 1860 to 1980. He shows a close relationship between the changing rate in the solar activities and the changing rate of the Earth’s tropospheric temperature. It can be noticed that the 11-year smoothed annual mean sunspot numbers (SSN) increased from about 45 during the year 1860 to about 80 for the year 1985, while the sea surface temperature increased by about 0.45 °C during the specified period. The correlation shows matching between the maxima and minima of the sea surface temperatures and the mean values of the sunspot number curves."
The observations that the terrestrial magnetic field is coupled in timing and intensity to planetary oxygen levels over hundreds of millions of years creates a first order problem of causative relay. The difficulty of attributing a causative mechanism without a solar scale energy source leaves few avenues to pursuing any viable options. Considering the level of predictive strength this model has already displayed in the solar magnetic forcing of climate and plate movement dynamics, leaves it at the present time the most viable candidate for this phenomena.
8<4.54 Observations of diapycnal upwelling within a sloping submarine canyon
Published: 26 June 2024
Bethan L. Wynne-Cattanach, Nicole Couto, Henri F. Drake, Raffaele Ferrari, Arnaud Le Boyer, Herlé Mercier, Marie-José Messias, Xiaozhou Ruan, Carl P. Spingys, Hans van Haren, Gunnar Voet, Kurt Polzin, Alberto C. Naveira Garabato & Matthew H. Alford
8<4.55 "The experiment site chosen was the Rockall Trough (Fig. 1), given its relatively flat, deep interior and steep sides. These characteristics enable a clear distinction between interior and boundary processes, which is essential for determining the driving forces behind the transformation of deep water. In particular, the study was conducted in a narrow, slope-incising canyon on the eastern side of the Rockall Trough. The canyon is 9-km wide at the mouth and 32-km long from the 2,900-m isobath to the tip of the eastern branch at the 1,200 m isobath. On average, the canyon walls rise 400 m above the thalweg. The canyon splits into a southwards and southeastwards branch around 54° 14.0′ N, 11° 57.1′ W. Our measurements focused on the southeastwards branch of the canyon."
8<4.56 "Here we show vigorous near-bottom upwelling across isopycnals at a rate of the order of 100 metres per day, coupled with adiabatic exchange of near-boundary and interior fluid. These observations were made using a dye released close to the seafloor within a sloping submarine canyon, and they provide direct evidence of strong, bottom-focused diapycnal upwelling in the deep ocean. This supports previous suggestions that mixing at topographic features, such as canyons, leads to globally significant upwelling. The upwelling rates observed were approximately 10,000 times higher than the global average value required for approximately 30 × 106 m3 s−1 of net upwelling globally."
8<4.57 "It has been hypothesized that upwelling may be confined to near-bottom regions within which the buoyancy flux perpendicular to the boundary must decrease to zero owing to the requirement of no flux through the boundary itself. The convergent turbulent buoyancy flux leads to upwelling near the boundary. Although the no-flux condition assumes no geothermal heat flux through the seafloor, inclusion of a geothermal heat flux reinforces upwelling at the boundary by warming deep waters from below."
8<4.58 "Previous estimates of upwelling near the bottom were smaller in magnitude but were observed over larger spatial and temporal scales than our experiment. The estimated average upwelling value required to maintain global deep-ocean density stratification is 1 × 10−2 m d−1 (ref. 9). In a fracture-zone canyon of the Mid-Atlantic Ridge, the magnitude of the up-canyon velocity was similar to that seen here. However, the shallower bathymetric slope led to a relatively smaller upwelling velocity of 1.7 m d−1 (ref. 7). Diapycnal upwelling in the Mid-Atlantic Ridge canyon is controlled by a decrease in the volume available for mixing as the canyon narrows (hypsometry)"
8<4.59 "Specifically, our results provide direct evidence in support of these studies’ key prediction: to overcome the substantial interior ocean downwelling implied by bottom-enhanced mixing, the net global upwelling of about 30 × 106 m3 s−1 requires the existence of much more rapid upwelling near the sloping seafloor."
8<4.591 Even if we were to ignore the possible, and more likely probable, contribution of a heat flux of geothermal convection, that has already been observed and its values measured, and that is currently circulating sea water into and out of the porous and faulted slopes that form the opposing ridge structures that run parallel along the length of the 40,000-kilometer (25,000 mile) oceanic ridge system. And, that includes a network of volcanoes, and generates new oceanic crust at the rate of 17 km3 per year and includes the massively larger by comparison Mid-Atlantic Ridge, that which, through convective motion, could contribute a heat engine conveyor component to the bottom water overturn process. Ignoring all of that, we can still assume a sizable contribution of thermal flux increases when the mantle thermal pulses periodically increase their volcanic activity.
The Mantle thermal pulses below the Mid-Atlantic Ridge that Enrico Bonatti Et al. observed and documented;
"show oscillations of ,3–4 Myr superimposed on a longer-term steady increase (and undoubtedly decrease) with time."
"They range along the VLS from 5.7% to 14.3%, with oscillations where the degree of melting appears to increase gradually and then decreases rapidly. These short-wavelength oscillations are superimposed on a long-term (.15Myr) trend of increasing (and undoubtedly decreasing) degree of melting with time"
8<4.592 If we consider these mantle thermal pulses once more in light of a 10,000 fold increase in the estimated overturn of bottom water volume;
8<4.593 ftp://ftp.nodc.noaa.gov/pub/data.nodc/woa/PUBLICATIONS/grlheat05.pdf
Warming of the world ocean, 1955–2003
S. Levitus, J. Antonov, and T. Boyer
National Oceanographic Data Center, NOAA, Silver Spring, Maryland, USA
Received 22 September 2004; revised 24 November 2004; accepted 8 December 2004; published 22 January 2005.
"Thus, a mean temperature change of 0.1 C. of the world ocean would correspond roughly to a mean temperature change of 100 C. of the global atmosphere if all the heat associated with this ocean anomaly was instantaneously transferred from the ocean to the atmosphere. This of course will not happen but this computation illustrates the enormous heat capacity of the ocean versus the atmosphere."
8<4.594 Would a miniscule increase in the global Mid-Ocean Ridge system's heat flux since the end of The Little Ice Age produce the observed warming that has occurred since then?
https://www.nature.com/articles/s41586-018-0614-0
Letter | Published: 24 October 2018
8<4.595 CO2 storage and release in the deep Southern Ocean on millennial to centennial timescales
J. W. B. Rae, A. Burke, L. F. Robinson, J. F. Adkins, T. Chen, C. Cole, R. Greenop, T. Li, E. F. M. Littley, D. C. Nita, J. A. Stewart & B. J. Taylor
Nature volume 562, pages 569–573 (2018)
Abstract
"The cause of changes in atmospheric carbon dioxide (CO2) during the recent ice ages is yet to be fully explained. Most mechanisms for glacial–interglacial CO2 change have centred on carbon exchange with the deep ocean, owing to its large size and relatively rapid exchange with the atmosphere1. The Southern Ocean is thought to have a key role in this exchange, as much of the deep ocean is ventilated to the atmosphere in this region2. However, it is difficult to reconstruct changes in deep Southern Ocean carbon storage, so few direct tests of this hypothesis have been carried out. Here we present deep-sea coral boron isotope data that track the pH—and thus the CO2 chemistry—of the deep Southern Ocean over the past forty thousand years. At sites closest to the Antarctic continental margin, and most influenced by the deep southern waters that form the ocean’s lower overturning cell, we find a close relationship between ocean pH and atmospheric CO2: during intervals of low CO2, ocean pH is low, reflecting enhanced ocean carbon storage; and during intervals of rising CO2, ocean pH rises, reflecting loss of carbon from the ocean to the atmosphere. Correspondingly, at shallower sites we find rapid (millennial- to centennial-scale) decreases in pH during abrupt increases in CO2, reflecting the rapid transfer of carbon from the deep ocean to the upper ocean and atmosphere. Our findings confirm the importance of the deep Southern Ocean in ice-age CO2 change, and show that deep-ocean CO2 release can occur as a dynamic feedback to rapid climate change on centennial timescales."
8<4.596 It would be this model's contention that "rapid (millennial- to centennial-scale) decreases in pH during abrupt increases in CO2, reflecting the rapid transfer of carbon from the deep ocean to the upper ocean and atmosphere" is indicative of a very sudden and sizable thermal source immediately adjacent, or more probable, just below, the carbon source. It's difficult to imagine something as passively mundane as solar thermal energy producing such a dramatic and rapid response from such a remote location. "abrupt" describes a mantle thermal pulse.
"What Rae et al. (2018) did is to look at deep-sea coral boron isotope data that track the pH — and thus the CO2 chemistry — of the deep Southern Ocean over the past forty thousand years. What they found is shown in the figure on the right. What it indicates is that as we moved from the Last Glacial Maximum (LGM) into the current inter-glacial, the pH of the deep Southern Ocean increased. This is consistent with the deep Southern Ocean sequestering CO2 during the LGM and then releasing it as we moved into the inter-glacial. As I mentioned at the beginning of this post, this is also the opposite of what we’d have expected if this region had been exchanging CO2 with the atmosphere during the LGM."
"So, this new work may have resolved one of the puzzles associated with the glacial cycles and demonstrates that a large amount of CO2 was indeed probably sequestered in the deep Southern Ocean during the glacial periods."
Ocean Warming: From the Surface to the Deep in Observations and Models
Durack, Gleckler, et al.
Oceanography; 9 Dec 2018;
"Although considerable work has conclusively shown significant warming in the upper (<700 m) ocean where the bulk of historical ocean temperature measurements are found (e.g., Rhein et al., 2013, and the section above on The Observing Network), and extending down to 2,000 m during the recent Argo period, there is now a growing consensus supported by numerous studies that changes are also occurring in the deeper global ocean (>2,000 m). Based on observations below 2,000 m, it is estimated that the global ocean has accumulated heat at a rate of 33 ± 21 TW over 1991 to 2010 (Desbruyeres et al., 2016). Two-thirds of this warming is occurring between 2,000 m and 4,000 m, albeit with large uncertainty, almost entirely owing to warming in the Southern Ocean in this depth range (see Sallée, 2018, in this issue). Below 4,000 m, the observations show a large meridional gradient in the deep warming rate, with the southernmost basins warming 10 times faster than the deep basins to the north (Figure 5A). While the warming below 4,000 m only accounts for one-third of the total warming below 2,000 m, the regional variability is lower, leading to greater statistical certainty in the abyssal changes (4,000 m to 6,000 m; Purkey and Johnson, 2010; Desbruyeres et al., 2016; Figure 5A)."
8<4.599 https://repository.library.noaa.gov/view/noaa/13231
Deep and abyssal ocean warming from 35 years of repeat hydrography.
Desbruyeres, Purkey, et al.
Geophysical Research Letters;
"The strongest warming rates are found in the abyssal layer (4000–6000 m), which contributes to one third of the total heat uptake with the largest contribution from the Southern and Pacific Oceans."
The Arctic Ocean warms from below
8<4.5914 "While geothermal heating appears to explain the warmer temperatures of CBDW, a fact remains that the salinity of the CBDW is higher by ∼0.02 psu than its presumed upstream source region in the Eurasian Basin. This requires a local source of salt and the most likely source is via shelf drainage of saline plumes, either as an ongoing steady renewal of approximately 0.5% of CBDW volume per year or as major episodic renewal events over decades or centuries (see discussions by Aagaard et al. [1985], Jones et al. [1995], Aagaard and Carmack [1994] and Rudels et al. [2012]). However, since geothermal heating and boundary convection are two independent processes they can, together, control the evolution of CBDW: shelf drainage and slope convection supplies higher salinity water and perhaps additional heat, whereas geothermal heating warms and stirs the bottom layer. While our work has focused on the Canada Basin, the observations of Björk and Winsor [2006] show geothermal heating to be a ubiquitous forcing element of the Arctic Ocean.""
8<4.5921
Climatic and tectonic drivers of late Oligocene Antarctic ice volume
B. Duncan, R. McKay, R. Levy, T. Naish, J. G. Prebble, F. Sangiorgi, S. Krishnan, F. Hoem, C. Clowes, T. Dunkley Jones, E. Gasson, C. Kraus, D. K. Kulhanek, S. R. Meyers, H. Moossen, C. Warren, V. Willmott, G. T. Ventura & J. Bendle
Nature Geoscience (2022)
8<4.5922 Abstract

8<4.5924 The mantle thermal pulses should now seem easily capable of very infinitesimal mantle that could produce a continual and varying short term thermal content into the ocean by way of the oceanic crust. These of course fit very nicely to documented thermal anomalies seen to occur as glaciated periods transitioned into inter-glacial periods.
8<4.5925 https://en.wikipedia.org/wiki/Younger_Dryas
"The change to glacial conditions at the onset of the younger Dryas in the higher latitudes of the Northern Hemisphere between 12,900–11,500 BP in calendar years has been argued to have been quite abrupt. in sharp contrast to the warming of the preceding Older Dryas interstadial. It has been inferred that its end occurred over a period of a decade or so, but the onset may have been faster." . . . . . .
. . . . . ."Various paleoclimatic records from ice cores, deep sea sediments, speleothems, continental paleobotanical data, and loesses show similar abrupt climate events, which are consistent with Younger Dryas events, during the terminations of the last four glacial periods. They argue that Younger Dryas events might be an intrinsic feature of deglaciations that occur at the end of glacial periods." . . . . . .
. . . . . . . "Measurements of oxygen isotopes from the GISP2 ice core suggest the ending of the Younger Dryas took place over just 40–50 years in three discrete steps, each lasting five years. Other proxy data, such as dust concentration, and snow accumulation, suggest an even more rapid transition, requiring about a 7 °C (13 °F) warming in just a few years.Total warming in Greenland was 10 ± 4 °C (18 ± 7 °F)."
8<4.6 This study from 2014 shows a convincing correlation between tree ring climate data and the solar magnetic record.
PLoS One. 2014; 9(4): e93504.
Published online 2014 Apr 1. doi: 10.1371/journal.pone.0093504
PMCID: PMC3972095
Tree-Ring Based May-July Temperature Reconstruction Since AD 1630 on the Western Loess Plateau, China
Huiming Song, Yu Liu, Qiang Li,1 Na Gao, Yongyong Ma, and Yanhua Zhang
Eryuan Liang, Editor
8<4.62 Abstract
"Tree-ring samples from Chinese Pine (Pinus tabulaeformis Carr.) collected at Mt. Shimen on the western Loess Plateau, China, were used to reconstruct the mean May–July temperature during AD 1630–2011. The regression model explained 48% of the adjusted variance in the instrumentally observed mean May–July temperature. The reconstruction revealed significant temperature variations at interannual to decadal scales. Cool periods observed in the reconstruction coincided with reduced solar activities. The reconstructed temperature matched well with two other tree-ring based temperature reconstructions conducted on the northern slope of the Qinling Mountains (on the southern margin of the Loess Plateau of China) for both annual and decadal scales. In addition, this study agreed well with several series derived from different proxies. This reconstruction improves upon the sparse network of high-resolution paleoclimatic records for the western Loess Plateau, China."
8<4.63 Method
"To identify climate-growth relationships for the Chinese pine on Mt. Shimen (MSM), a correlation analysis was performed for monthly mean temperature, monthly total precipitation and PDSI over the previous and current years of tree growth. The model stability and reliability were assessed using a split-sample method in which the model was divided into two subsets of equal length [20], [21].The Pearson correlation coefficient (r), the positive RE (reduction of error) and CE (coefficient of efficiency) are the items to evaluate the results. Spatial correlations were used to assess the representativeness of our reconstruction by using the KNMI Climate Explorer (Royal Netherlands Meteorological Institute; http://climexp.knmi.nl)."
"The positive RE and CE results indicated that the reconstruction contributes unique paleoclimatic information. In addition, Figure 5a showed that the reconstruction closely tracked the observed temperature."
8<4.64 Temperatures on Mt. Shimen
"Figure 5b shows the reconstructed May-July temperature variations since AD 1630 and its 20-year low-pass filter on Mt. Shimen (MSM) This series shows interannual to multi-decadal variability (Fig. 5b). The long cooling period from AD 1640 to 1720 corresponds with well-known periods of solar minima (Maunder Minimum) during AD 1645–1715 [19]. Two obvious cool periods that occurred during the 1880s–1900s and the 1930s–1960s coincide with the 1900 minimum (AD 1880–1900) and a slight decrease in sun activity from AD 1940–1970, respectively [27]. The cool period during the reconstruction matched all solar minima except the Dalton minimum (AD 1800–1820), implying that solar forcing likely played an important role in past climate change for MSM. The longest warming trend occurred during AD 1720s–1810s and was followed by a mild climate for approximately 50 years. In addition, the filtered reconstruction indicates that the warming since AD 1970s is not significant and does not exceed the natural temperature variations that occurred over the past 400 years. AD 1928 stands out as an unusually warm year and corresponds with the drought event in AD 1928–1929, which has been noted as a dominant feature of the drought record throughout northern China[28], [29]. Additionally, the warm year of AD 2000 is consistent with the severe drought that occurred in China [9]."
-----------------------------------------------------------------------------------------------------------------
Page 9
Evidence of a
correlated and cyclical
behavior of
Crustal movement and climate
-----------------------------------------------------------------------------------------------------------------------------------------------------------------------------------------------------------------
9<4.65 Previously, this model showed us a remarkable prediction of the correlation of solar magnetic 14C proxy data, climate data (Medieval Warm Period and Little Ice Age) and Japanese earthquake records beginning in the Edo period and continuing up to the present time. Thus showing the model's predictive abilities to provide a simple chain of causative relay as the Earth's field generator responded to an increase in solar magnetic forcing and then relayed its thermal response into the surrounding mantle and initiating the corresponding seismic energies and accompanying transient climate.
9<4.66 Some might wonder if that correlation could be just a coincidence and unlikely to be found to have happened previously at a different time in the geologic record.
9<4.7 This model proposes that the energy responsible for subduction is gravitational potential energy (GPE) within the crust, which is put into motion as kinetic energy when the mantle periodically subsides. This variable GPE will slowly increase as the crust incrementally becomes compressed by the subsiding mantle as it responds to the thermal contraction of the core. This GPE is present, to some degree, at all times in the crust but is at its highest potential when the mantle's subsidence outpaces the trenches' ability to process the subducting crust at a rate high enough to keep pace with the mantle's movement towards the core. As these two metrics diverge the GPE in the crust will increase. When the core's thermal cycle changes to outward displacement the GPE is then slowly reduced as the outward movement of the mantle increasingly re-supports the crust.
9<4.71 This cycle however is punctuated by distinct periods when oceanic crust is produced. These periods only occur during the outward displacement portion of the cycle as the divergent oceanic plate sections are moved apart by the displacing mantle. These active spreading periods will be shown to correlate to climate warm periods, and alternately, it will be shown that the climate cools when the active seafloor spreading periods slow or in rare instances; come to an end.
9<4.72 In the study below the research strongly indicates that this link is evident in the geologic record. The seafloor spreading appears to have almost completely halted or slowed to barely discernible levels for 250 million years. At which time the Earth entered a period known as Snowball Earth.
9<4.73 https://www.researchgate.net/publication/223745786_Evidence_and_implications_for_a_widespread_magmatic_shutdown_for_250_My_on_Earth
Evidence and implications for a widespread magmatic shutdown for 250 My on Earth
Earth & Planetary Sci. Letters 282, (2009): 294-298 Condie, K. C., O’Neill, C. and Aster, R.
Abstract
9<4.74 "Analysis of the global distribution of U/Pb ages of both subduction-related granitoids and of detrital zircons suggests that a widespread reduction in magmatic activity on Earth beginning about 2.45 Ga and lasting for 200–250 My. . . . . . .There is little Nd or Hf isotopic evidence to support significant additions to the continental crust at convergent plate margins between 2.45 and 2.2 Ga. . . . . . . Oxygenation of the atmosphere at 2.4 Ga followed by widespread glaciation at 2.4–2.3 Ga also may be related to the initiation of the global magmatic lull. We suggest that an episodic mantle thermal regime, during which a large part of the plate circuit effectively stagnates, may explain the 250-My magmatic age gap on Earth and a remarkable feature of the Paleoproterozoic record."
9<4.75 Conclusions
"The distribution of U/Pb zircon ages from both subduction-related granitoids and detrital sediments shows a pronounced and robust minimum between 2.45 and 2.2Ga .Furthermore, there is a sparsity of greenstones and subduction-related granitoids, as well as evidence for juvenile continental crust in this 250-My time window. We hypothesize that this reflects a globally significant period of cessation or slowdown global magmatism and perhaps in plate tectonics".
9<4.76 http://www.nmt.edu/news/3243-tech-professors-research-making-international-headlines
"SOCORRO, N.M. – New Mexico Tech geophysics professor Dr. Kent Condie has been making headlines around the world in recent weeks."
9<4.77 "Condie is the first author of an academic paper in Earth and Planetary Science Letters that purports to show evidence that tectonic activity slowed to a crawl for more than 200 million years, creating a scenario some scientists call “snowball Earth.” Condie’s paper has two co-authors: Dr. Craig O’Neill of Macquarie University in Sydney, Australia and Dr. Rick Aster, also of New Mexico Tech. The trio analyzed data from many sources in geology, geochemistry and geochronology."
9<4.78 This very significant and "widespread magmatic shutdown" event described by Condie et.al. above would appear at a very specific transitional period of the already observed mantle thermal pulses of Bonatti et. al. Specifically, that when the Sun's solar magnetic energy generation output ended abruptly, the final outward period of the current mantle thermal pulse would have ended also. The Sun then entered a +/- 250 million year solar magnetic energy level hiatus. As the Earth's crust slowly distributed the last of its gravitational potential energy and its resultant latent plate movement, the planet continued to cool to the point that was described by Condie et.al. as a "widespread magmatic shutdown". This period was called the Huronian glaciation and is considered one of the most extensive glaciated periods often referred to as a Snow Ball Earth.
9<4.79 So here we have two of the three predicted correlated phenomena. In this case, the stagnant plate movement and climate that both exhibit the historical, all time lowest observed energy state of both climate and tectonic plate energies. What about the Solar/planetary magnetic field component? The model would predict that the Solar magnetic field energies would be very low, and the corresponding Planetary field would be so low for so long that there would be no significant variable mantle displacement and it's accompanying strain energy thermal warming of the oceans and atmosphere.
9<4.791 In this study below the researchers have sampled the lowest recorded planetary magnetic field strength less then 100 Ma after another major global glaciation that took place during the Ediacaran. "The Marinoan glaciation, was a period of worldwide glaciation. Its beginning occurred no earlier than 654.5 Ma (million years ago). It ended approximately 632.3 ± 5.9 Ma during the Cryogenian period. This glaciation possibly covered the entire planet, in an event also referred to as a Snowball Earth." -https://en.wikipedia.org/wiki/Marinoan_glaciation
9<4.792 And was just after the; "Gaskiers glaciation a period of widespread glacial deposits that lasted under 340 thousand years, between 579.63 ± 0.15 and 579.88 ± 0.44 million years ago i.e. late in the Ediacaran Period — making it the last major glacial event of the Precambrian. It was also the last and the shortest of at least three major ice ages in the Neoproterozoic era. It is assumed that, in contrast to the Sturtian and Marinoan periods, it did not lead to global glaciation." -https://en.wikipedia.org/wiki/Gaskiers_glaciation
9<4.793 https://www.nature.com/articles/s41561-018-0288-0
Article Published: 28 January 2019
Young inner core inferred from Ediacaran ultra-low geomagnetic field intensity
Richard K. Bono, John A. Tarduno, Francis Nimmo & Rory D. Cottrell
Nature Geoscience volume 12, 143–147 (2019)
9<4.794 "Here we present palaeointensity data from the Ediacaran (~565 million years old) Sept-Îles intrusive suite measured on single plagioclase and clinopyroxene crystals that hosted single-domain magnetic inclusions. These data indicate a time-averaged dipole moment of ~0.7 × 1022 A m2, the lowest value yet reported for the geodynamo from extant rocks and more than ten times smaller than the strength of the present-day field."
9<4.795 The model suggests that a correlation will be made between solar/planetary magnetic field strength, tectonic plate movement and climate. The first being the solar magnetic energy increase at the end of the Little Ice Age that aligns to the greater quantity and severity of Japanese earthquakes. Marking a distinct period of a transition of the phenomena from a lower to a higher energy state. And now the same model appears it can plausibly predict and show the phenomena in an energy correlation of the lowest detected planetary magnetic field energy or the lowest detected tectonic plate kinetic energy occurring when the planet endured two of the largest and coldest known climate periods in Earth's history.
9<4.796 We can imagine that moment +/- 250 million years later when the solar magnetic energy output increased back to the required levels to restart those higher energy Bonatti mantle thermal pulses once again, producing strain energy displacement of the mantle and releasing thermal content at the crust mantle boundary and the newly activated divergent boundaries.
9<4.797 So now we can see that the solar magnetic generator's variable cycle of operational quantity and duration would fit nicely to this phenomenon as well as it does to those more mundane cycles discussed earlier that were attributed to the general plate movement idea of this model.
9<4.798 What does plate movement look like at the transition periods where plate energies wind-down to 0 and the solar magnetic energy comes back up suddenly to levels that produce basaltic magma province size eruptions like those that began forming the Atlantic ocean basin?
9<4.799 https://www.science.org/doi/10.1126/sciadv.abo0866
Reduced plate motion controlled timing of Early Jurassic Karoo-Ferrar large igneous province volcanism
Micha Ruhl, Stephen P. Hesselbo, Hugh C. Jenkyns, Weimu Xu., Ricardo L. Silva, Kara J. Matthews, Tamsin A. Mather, Conall Mac Niocaill, And James B. Riding
SCIENCE ADVANCES 9 Sep 2022 Vol 8, Issue 36
DOI: 10.1126/sciadv.abo0866
9<4.7910 Abstract
"Past large igneous province (LIP) emplacement is commonly associated with mantle plume upwelling and led to major carbon emissions. One of Earth’s largest past environmental perturbations, the Toarcian oceanic anoxic event (T-OAE; ~183 Ma), has been linked to Karoo-Ferrar LIP emplacement. However, the role of mantle plumes in controlling the onset and timing of LIP magmatism is poorly understood. Using global plate reconstruction models and Lower Toarcian sedimentary mercury (Hg) concentrations, we demonstrate (i) that the T-OAE occurred coevally with Karoo-Ferrar emplacement and (ii) that timing and duration of LIP emplacement was governed by reduced Pangean plate motion, associated with a reversal in plate movement direction. This new model mechanistically links Earth’s interior and surficial processes, and the mechanism is consistent with the timing of several of the largest LIP volcanic events throughout Earth history and, thus, the timing of many of Earth’s past global climate change and mass extinction events."
9<4.7911 "The Siberian Traps, the Karoo-Ferrar, the North Atlantic Igneous Province, and the East African Rift LIP regions all show a substantial reduction in local plate velocities and an associated reduction in local total plate movement in the millions of years leading up to LIP emplacement"
9<4.7912 So, we can see in the paper above the plate movement of this model describes and predicts perfectly the conditions described in the just published research where GPE is dispersing to the point of cessation of plate movement in one specific direction several million years before the initiation of a large igneous province and the resultant plate movement in the opposite direction.
Where have we also seen this direction reversing plate behavior described in the available research?
9<4.7913
https://www.researchgate.net/publication/309737839_Rapid_conversion_of_an_oceanic_spreading_center_to_a_subduction_zone_inferred_from_high-precision_geochronology
Rapid conversion of an oceanic spreading center to a subduction zone inferred from high-precision geochronology
Timothy E. Keenan (a), John Encarnación (a1), Robert Buchwaldt (b), Dan Fernandez (c), James Mattinson(d), Christine Rasoazanamparany (e), and P. Benjamin Luetkemeyer(a)
Author Affiliations
aDepartment of Earth and Atmospheric Sciences, Saint Louis University, St. Louis, MO 63108;
bDepartment of Earth and Environment, Boston University, Boston, MA 02215;
cSchlumberger-WesternGeco, Geosolutions-Interpretation, Houston, TX 77042;
dDepartment of Earth Science, University of California, Santa Barbara, CA 93106
eDepartment of Geology and Environmental Earth Science, Miami University, Oxford, OH 45056
Edited by W. G. Ernst, Stanford University, Stanford, CA, and approved October 11, 2016 (received for review June 20, 2016)
9<4.7914 Significance
"Subduction, the process by which tectonic plates sink into the mantle, is a fundamental tectonic process on Earth, yet the question of where and how new subduction zones form remains a matter of debate. In this study, we find that a divergent plate boundary, where two plates move apart, was forcefully and rapidly turned into a convergent boundary where one plate eventually began subducting. This finding is surprising because, although the plate material at a divergent boundary is weak, it is also buoyant and resists subduction. This study suggests that buoyant, but weak, plate material at a divergent boundary can be forced to converge until eventually older and denser plate material enters the nascent subduction zone, which then becomes self-sustaining."
9<4.7915 Abstract
"Where and how subduction zones initiate is a fundamental tectonic problem, yet there are few well-constrained geologic tests that address the tectonic settings and dynamics of the process". . . . . . . . . . . "In the western Philippines, we find that oceanic crust was less than ∼1 My old when it was underthrust and metamorphosed at the onset of subduction in Palawan, Philippines, implying forced subduction initiation at a spreading center. This result shows that young and positively buoyant, but weak, lithosphere was the preferred site for subduction nucleation despite the proximity of other potential weak zones with older, denser lithosphere and that plate motion rapidly changed from divergence to convergence".
The divergent boundary "was forcefully and rapidly turned into a convergent boundary".
9<4.7916 These observations have consistently shown that the tectonic plates behave in the manner consistent with the predictions outlined by this model.
9<4.8 These phases between the inward and outward displacement of the mantle should be easily observable in the geologic record. We should be able to see specific areas of the earth's crust where repeated periods of extensional movement was interrupted by shorter periods of compressive shortening.
https://www.researchgate.net/publication/249546906_Evidence_from_the_Gediz_Graben_for_episodic_two-stage_extension_in_western_Turkey
9<4.81
Evidence from the Gediz Graben for episodic two-stage extension in western Turkey
Journal of the Geological Society 156(3):605-616 · June 1999
Erdin Bozkurt, Middle East Technical University | METU · Department of Geological Engineering
A. KOCYIDIT, affiliated with Middle East Technical University (Çankaya, Turkey)
H. YUSUFODLU
9<4.82 Abstract
"Western Turkey falls within a wide belt of NNE-SSW-directed, active continental extension, generally similar to the Basin and Range Province of the USA. It comprises a zone of WNW-ESE-trending major grabens. Detailed study of the Gediz graben reveals two contrasting infills that represent two distinct extensional stages, separated by a short phase of compression. The older infill consists of an 800 m thick, folded and exhumed continental sedimentary sequence with intercalated calc-alkaline volcanics. It accumulated in a basin formed in the northern hanging wall of a detachment fault during Miocene-early Pliocene times. The younger infill rests on the older infill with angular unconformity and consists of about 170 m of undeformed, terraced continental sediments and basaltic lavas. It accumulated during Plio-Quaternary times in an asymmetric graben bounded by step-like normal faults. This later extension was probably triggered by the commencement of sea-floor spreading along the Red Sea in early Pliocene times and has persisted to the present. Almost all the grabens in western Turkey are best explained by this episodic, two-stage graben model with an intervening phase of short-term compression, which differs from the progressive evolution inferred for the Basin and Range Province of the USA."
9<4.83 Remember, we are talking about an expansion total at the current divergent boundaries of maybe 20 cm a year out of almost 40075.16 kilometers (24901.55 miles) of the Earth’s circumference. This, while the current inventory of GPE is slowly unloading as it disperses into the convergent boundaries, and raising various mountain ranges like the Himalayas to increasing heights.
-----------------------------------------------------------------------------------------------------------------
Page 10
Evidence that the
model accurately predicts
The observations of crustal behavior
------------------------------------------------------------------------------------------------------------------------------------------------------------------------------------------------------------------
10<5.01 Imagining the Earth with one single belt of sea floor around the equator (above) with one end considered attached, immovable. The other end just a short distance away unconnected. Then we apply the thermal increase that displaces the mantle and extends the crust. We can now see the gap between the plate ends open a given degree.
10<5.02 As seen in the examples shown previously, the magnetic field is in a constant state of change, varying in duration and intensity. At the other end of this chain of events is the crust being subjected to shear forces imposed by a displacing or subsiding mantle. I have built many things involving laminating materials into radii shapes. These are unlike materials such as metals and plastics of various densities and thicknesses. The material on the inside is able to deform in radius with little or no structural compromise, but the outside material must have either resilient qualities or a differential of allowable movement, which is the ability to move independently to compensate for the uneven rate of movement between the two layered composites.
10<5.1 The mantle/crust boundary is similar;
http://www.dst.uniroma1.it/sites/default/files/doglioni/E6-15-03-13-TXT.aspx.html
MANTLE DYNAMICS AND PLATE KINEMATICS
Carlo Doglioni, La Sapienza University, Rome, Italy
Roberto Sabadini, University of Milan, Italy
10<5.11 ". . . . . none of the proposed models of mantle convection can account for the simpler pattern in plate motion we observe at the surface, nor has a unique solution been proposed for how material in the mantle convects. At the moment there is no way to link mantle dynamics and plate kinematics at the surface, considering that the mantle and lithosphere are detached. The Atlantic and Indian ridges are in fact moving apart with respect to Africa, proving not to be fixed both relative to each other and relative to any fixed point in the mantle. This evidence confirms that ocean ridges are decoupled from the underlying mantle."
"The Atlantic and Indian ridges are in fact moving apart with respect to Africa, proving not to be fixed both relative to each other and relative to any fixed point in the mantle"
10<5.12 This observation alone defines the mantle dynamics described by this model. The differential of allowable movement is clearly described in that statement. Add this to the earlier observation of mantle oscillations;
https://www.academia.edu/38174615/Mantle_thermal_pulses_below_the_Mid_Atlantic_Ridge_and_temporal_variations_in_the_formation_of_oceanic_lithosphere
10<5.13 Mantle thermal pulses below the Mid-Atlantic Ridge and temporal variations in the formation of oceanic lithosphere
10<5.14 "A 20-Myr record of creation of oceanic lithosphere at a segment of the central Mid-Atlantic-Ridge is exposed along an uplifted sliver of lithosphere. The degree of melting of the mantle that is upwelling below the ridge, estimated from the chemistry of the exposed mantle rocks, as well as crustal thickness inferred from gravity measurements, show oscillations of ,3–4 Myr superimposed on a longer-term steady increase with time. The time lag between oscillations of mantle melting and crustal thickness indicates that the solid mantle is upwelling at an average rate of ,25mmyr, but this appears to vary through time."
10<5.15 The evidence for the current outward displacement of the mantle is well supported by these two statements, and there is more to come.
-----------------------------------------------------------------------------------------------------------------
Page 11
A model of
Seafloor dynamics
------------------------------------------------------------------------------------------------------------------------------------------------------------------------------------------------------------------
11<5.2 If the Earth’s crust was put under a small amount of core/mantle thermal displacement it would stretch then separate at its weakest point. The number of separations would be dependent on the amount of the differential of allowable movement. If the crust had unlimited strength one separation would suffice, but its numerous weaknesses are witness to the shear resistance the crust has to the mantle. All the divergent plate boundaries around the world began with a tension break like this.
11<5.21 During the mantle's outward displacement the oceanic crust that extends unbroken from its continental attachment point out to its divergent plate boundary will remain so until the point in time when its structurally weakest areas will fracture. This fracturing is based on the increased frictional drag or shear forces that are increasingly applied to the plate from its own periodically increasing metric expanse extending out to its opposing edge.
11<5.23 All oceanic plates will separate from this increasing shear stress. The Pacific plate did so long ago along its western continental margin and then established convergent boundaries at many of these fractures during following periods of contraction. And, in due time with greater plate width, the Atlantic will do the same.
11<5.3 This means that when the mantle displaces outward; any oceanic plate that cannot relieve the tension derived from either its own extreme plate width, or, due to it having its opposing plate ends held by subduction, as in having been overran and held by a continent or another oceanic plate, the plate will fracture at its weakest point. And of course, as described previously, these newly created divergent plate boundaries can be converted at a later time into a convergent plate boundary when the mantle once again begins to subside and the Earth's crust will need to relieve the growing GPE through the compression relieving mechanisms known as convergent boundaries.
11<5.31 "Eventually the magnetic field energy lowers and the outer core contracts placing the crust in compression against the most recent deposits of seafloor. The first subductions probably began during that time long ago when all that existed was proto crust beneath a shallower ocean. When the compression reached a critical level a compression ridge formed at one of the weaker divergent boundaries. As it failed under the increasing pressures it became a convergent boundary as one edge subducted under the other."
11<5.33 Rapid conversion of an oceanic spreading center to a subduction zone inferred from high-precision geochronology
Timothy E. Keenan (a), John Encarnación (a1), Robert Buchwaldt (b), Dan Fernandez (c), James Mattinson(d), Christine Rasoazanamparany (e), and P. Benjamin Luetkemeyer(a)
Author Affiliations
aDepartment of Earth and Atmospheric Sciences, Saint Louis University, St. Louis, MO 63108;
bDepartment of Earth and Environment, Boston University, Boston, MA 02215;
cSchlumberger-WesternGeco, Geosolutions-Interpretation, Houston, TX 77042;
dDepartment of Earth Science, University of California, Santa Barbara, CA 93106
eDepartment of Geology and Environmental Earth Science, Miami University, Oxford, OH 45056
Edited by W. G. Ernst, Stanford University, Stanford, CA, and approved October 11, 2016 (received for review June 20, 2016)
11<5.34 Significance
"Subduction, the process by which tectonic plates sink into the mantle, is a fundamental tectonic process on Earth, yet the question of where and how new subduction zones form remains a matter of debate. In this study, we find that a divergent plate boundary, where two plates move apart, was forcefully and rapidly turned into a convergent boundary where one plate eventually began subducting. This finding is surprising because, although the plate material at a divergent boundary is weak, it is also buoyant and resists subduction. This study suggests that buoyant, but weak, plate material at a divergent boundary can be forced to converge until eventually older and denser plate material enters the nascent subduction zone, which then becomes self-sustaining."
11<5.35 Abstract
"Where and how subduction zones initiate is a fundamental tectonic problem, yet there are few well-constrained geologic tests that address the tectonic settings and dynamics of the process". . . . . . . . . . . "In the western Philippines, we find that oceanic crust was less than ∼1 My old when it was underthrust and metamorphosed at the onset of subduction in Palawan, Philippines, implying forced subduction initiation at a spreading center. This result shows that young and positively buoyant, but weak, lithosphere was the preferred site for subduction nucleation despite the proximity of other potential weak zones with older, denser lithosphere and that plate motion rapidly changed from divergence to convergence".
11<5.36 The divergent boundary "was forcefully and rapidly turned into a convergent boundary". This does not describe the current model's passive process: "of other potential weak zones with older, denser lithosphere"
"young and positively buoyant, but weak, lithosphere was the preferred site for subduction nucleation"
"plate motion rapidly changed from divergence to convergence".
-----------------------------------------------------------------------------------------------------------------
Page 12
A model of
Convergent dynamics
------------------------------------------------------------------------------------------------------------------------------------------------------------------------------------------------------------------
Image above courtesy of USGS
12<5.4 Let’s apply this mechanism to one of these newly converted "divergent/convergent" boundaries like the one in the study above; one that will eventually develop a string of volcanic Island Arcs.
12<5.41 When the core's thermal cycle causes the mantle to subside, this “thermal contraction” will produce gravitational potential energy in the crust that in turn provides the compression to advance the ocean crust under the overlying continental-oceanic crust. This process would undoubtedly force the overlying crust to be lifted up. At a point behind the convergent zone determined by the structural deficiencies of the overriding plate, the overriding plate will fracture.
12<5.42 These fractures are directly correlated to the subducting plate being forced under the overriding plate. When the “thermal expansion” portion of the thermal cycle begins again, the Earth’s crust is slowly radially extended, opening all divergent plate boundaries to the inflow of fresh magma, including these newly created and fractured back-arc basin spreading centers.
12<5.43 The deep oceanic trenches are put into tension when the upper mantle is periodically extended to unusual levels of expansion that removes all or most of the GPE in those particular crustal plate sections. These are periods when the mantle once again begins to slowly displace outward after a longer than usual period of lower magnetic field strength where the compression in the crust has had the additional time to largely dissipate and can thus allow tension to form in the larger continental and oceanic plates.
12<5.44 The resulting tension causes the oceanic crust to pull against its fixed position in the subducted convergent boundary zone, pulling back on the edge of the oceanic crust where it is bent downward. This tension force is proportional to the distance to the divergent plate boundary. A section of oceanic crust that is 1000 km between the trench and mid ocean ridge will have twice the tension at the trench edge and twice the allowable movement at the divergent boundary; during expansion, as one that is 500 km.
Image above used and modified by this author was furnished through and in no way endorsed by://www.geomapapp.org using Global Multi-Resolution Topography (GMRT) Synthesis,
Ryan, W. B. F., S.M. Carbotte, J. Coplan, S. O'Hara, A. Melkonian, R. Arko, R.A. Weissel, V. Ferrini, A. Goodwillie, F. Nitsche, J. Bonczkowski, and R. Zemsky (2009), Global Multi-Resolution Topography (GMRT) synthesis data set, Geochem. Geophys. Geosyst., 10, Q03014, doi:10.1029/2008GC002332.
12<5.45 This is why I believe the Mariana trench (A) (Image above) is the deepest trench in the world; it has the most applied tension because its latitudinal ocean crust section of that area of the Pacific Plate is unusually wide causing the arc shape to form with an unusually deep convergent trench. More lateral distance = more applied tension. More lateral distance = more divergent boundary movement (Fig. 1).
12<5.5 But there is an additional element to the Mariana tension mechanism; around 28 million years ago the mid-ocean ridge and a portion of the Pacific Plate itself on its eastern plate margin had been overrun and held by the North American continent (Fig. 2), resulting in the trench opening being spread wider and deeper than it would be with just having a long expanse of oceanic crust terminating at the Mid-Ocean Ridge (Fig. 1). This was for a very short period of time that occurred immediately after the Pacific plate divergent boundary followed the Farallon into the subduction zone. And it ended when the next mantle thermal pulse began and slowly pulled the plate end back to the west. Another dynamic was introduce at this time when the northwest direction of the Pacific plate was applied to this boundary as the pacific plate subducted and then retracted while moving in the northwest direction. This would undoubtedly produce a extraordinary result as the Pacific plate end laterally excavated the area beneath N.A. plate margin as the plate withdrew and established the currently observed San Andres margin plate boundary dynamics.
12<5.51 When just one end of the plate is held by the trench the allowable movement at the divergent boundary will moderate the tension in an ebb and flow of tension and movement (Fig. 1) as the crust slides to relieve the tensile stresses caused from the underlying shear forces that occur as the mantle displaces outward.
12<5.52 But in the Pacific the allowable movement mechanism became almost completely eliminated in this plate section when the Pacific Plate was overran and held by the North American land mass (Fig. 2).
12<5.54 It is this gap that fills with magma creating new seafloor as the allowable movement satisfies the building tension. With this stress relieving element removed the trench is subjected to larger and longer periods of tension. More applied tension without allowable movement = wider and deeper trenches.
-----------------------------------------------------------------------------------------------------------------
Page 13
A model for
Extensional dynamics
------------------------------------------------------------------------------------------------------------------------------------------------------------------------------------------------------------------
Image below used and modified by this author was furnished through and in no way endorsed by://www.geomapapp.org using Global Multi-Resolution Topography (GMRT) Synthesis,
Ryan, W. B. F., S.M. Carbotte, J. Coplan, S. O'Hara, A. Melkonian, R. Arko, R.A. Weissel, V. Ferrini, A. Goodwillie, F. Nitsche, J. Bonczkowski, and R. Zemsky (2009), Global Multi-Resolution Topography (GMRT) synthesis data set, Geochem. Geophys. Geosyst., 10, Q03014, doi:10.1029/2008GC002332.
Data doi: 10.1594/IEDA.0001000, through http://creativecommons.org/licenses/by-nc-sa/3.0/us/
13<5.56 In the S.W. United States there is the Basin and Range area (B, inside black outline), the area's lateral displacement varies between 60 to 300 km since the beginning of the extension in the early Miocene. The Miocene Epoch was 23.03 to 5.3 million years ago. Currently there is no clear and concise explanation for this event.
13<5.57 What is remarkable though, is that the particular latitudinal section of Pacific oceanic seafloor that had been partially subducted, or more accurately, overran by the N. American continent, extends all the way to the Mariana Trench (A) in the western Pacific.
13<5.58 Interesting isn't it. The deepest trench in the world is on one end; and the largest example of extensional processes is on the other.
13<5.59 What is also interesting and a relevant development is this recent paper that reveals a shallow subduction regime has been modeled;
13<5.510 https://confit.atlas.jp/guide/event/jpguagu2017/subject/SIT29-01/detail?lang=en
New perspectives on East Asia geodynamics from the crust to the mantle
Tue. May 23, 2017 9:00 AM - 10:30 AM A03 (Tokyo Bay Makuhari Hall)
convener:Timothy B Byrne(University of Connecticut), Gaku Kimura (Tokyo University of Marine Science and Technology), Jonny Wu (University of Houston), Kyoko Okino (Atmosphere and Ocean Research Institute, The University of Tokyo), Chairperson:Tim Byrne (University of Connecticut), Chairperson:Okino Kyoko (Atmosphere and Ocean Research Institute, The University of Tokyo)
13<5.511 "Seismic tomography has revealed enigmatic stagnant slab anomalies under Japan, Korea and NE China (i.e. the Japan slab). The stagnant slabs flatten near the mantle transition zone around ~410 to 660 km depths and extend >2000 km westward from the NW Pacific subduction zones. The location of the outboard stagnant slabs far inland under Eurasia cannot be explained by slab rollback alone and pose a challenge to our current understanding of subducted slab dynamics, in which slabs sink vertically over time with minimal lateral motion."
13<5.512 "Our reconstruction implies the Japan slabs moved laterally westwards within the upper mantle and transition zone after subduction at near-plate tectonic rates (~2 cm/yr over 50 Ma), indicating a greater lateral mobility of slabs within the upper mantle and transition zone than previously recognized."
13<5.52 Now, if we could imagine that this type of subduction described above is not a rare occurrence at all, and instead is a very common form of subduction. And if we could further imagine that one of these very shallow subducted plates was again the Pacific, but instead, this time, it was the opposite subducted edge on the Pacific plate's eastern margin, and that it had simply followed and then subducted alone with the Farallon plate that had just prior to this been completely overran up to when its divergent boundary was consumed under the overriding continent, with the Pacific plate simply following and then moving under the edge of the Farallon plate.
13<5.521 https://www.iris.edu/hq/NSFProposal/Volume2/Lith.pdf
Lithospheric Layering in the North American Craton
Huaiyu Yuan (Berkeley Seismological Laboratory),
Barbara Romanowicz (Berkeley Seismological Laboratory)
13<5.522 "Recent receiver function studies detect structural boundaries under continental cratons at depths too shallow to be consistent with the lithosphere-asthenosphere boundary (LAB) as inferred from seismic tomography and other geophysical studies. Using the new results from our regional surface wave tomographic inversion for the North American upper mantle shear wave structure, we show (Figure1) that changes in the direction of azimuthal anisotropy with depth reveal the presence of two distinct lithospheric layers throughout the stable part of the North American (NA) continent [Yuan and Romanowicz, 2010]."
. . . . ."American craton may be exceptionally simple, the application of this tool to other continents should provide further insights on the assembly and evolution of cratons worldwide."
13<5.523 If we look back to the description this model made earlier of the; "initial development and evolution of plate tectonics" we can see a very similar scenario described, in fact an almost identical scenario. Multiple layers of oceanic crust subducted one after another, each time after the plate had broke during the intermittent outward displacement periods. We can imagine that the plate would have been loaded with considerable tension before it was broken free, and may have imposed these tension forces as a great shear stress into the overriding crust, even stretching it out as it broke the over-riding crust into many parallel segments.
13<5.524 And now, if we reexamine the Basin and Range Province and its relationship to the Pacific plate we could deduce a cause for the extension of that area of continental crust.
13<5.53 The eastern side of Pacific shows distinct sections of seafloor that have defined fault slip edges; the Mendocino to the north, followed by in consecutive order to the south; Murray, Molokai and Clarion, that have facilitated the crustal section's differential of movement. After a series of possibly several cycle periods the model has the Pacific Plate over ran and held by the North American continent and the Farallon plate that lies directly beneath it. With the divergent boundary movement eliminated, the boundary compression in these plate sections was reduced as the existing GPE slowly drained away into the convergent boundaries on the western margin of the plate.
13<5.54 As the cycle then changed to thermal expansion of the core with its accompanying mantle displacement occurring directly below the Basin and Range, the Pacific plate section was loaded with slowly increasing tension, and due to its inability to move through tension relieving mechanisms such as the Pacific Ridge does currently at various locations in the Southern Hemisphere, this tension was passed on to the overriding plate that kept it from relieving its growing tensional strain. That boundary's subduction under the edge of the N. American continent eliminated all or most of the boundary's ability to function as a standard tension relieving mechanism like the Atlantic MOR does. The Basin and Range was pulled instead as the Pacific plate moved north and excavated itself out from under the overlying continent, possibly leaving the Central Valley a void to drop down into, while the Mariana Trench was pulled toward the east. This is clearly observed in the images below.

13<5.55 The Mariana Back-Arc clearly exhibits the evidence that it has behaved as a tension relieving mechanism, leaving its series of progressive compression ridges marking the periods when the back-arc expansion had ended and the various boundaries were then subjected to prolonged periods of crustal compression. While the Basin and Range clearly shows the extensional Horst and Grabin terrain exhibiting normal faults of opposite dip that occur in pair with parallel strike lines.
-----------------------------------------------------------------------------------------------------------------
Page 14
A model of
Convergent boundary dynamics
------------------------------------------------------------------------------------------------------------------------------------------------------------------------------------------------------------------
14<5.56 This 3-D sketch (left) shows a cross-section of the Mariana Arc with some of its main structures and features. The numbers represent the speed of sound in the various layers beneath the arc measured by the precise timing arrivals from earthquakes and man-made sources. Higher velocities represent more dense material. Note that there is old ocean crust descending in the trench at the same time new crust is forming along the island arc (from deep melting of the down going plate) and in the back-arc spreading center (from extension behind the arc). Modified from Plate 2 of Fryer and Hussong (Proceedings of the Deep Sea Drilling Project, Leg 60, pp. 45-55, 1981). Image and text courtesy NOAA Ocean Explorer.
14<5.6 When the core's thermal cycle changes once again from expansion to thermal contraction and the upper mantle moves back towards the core; most isolated areas of tension, like which was described above with the Pacific, is slowly replaced by compression; incrementally increasing the crust's inventory of GPE derived compression that is then slowly released against the planet's divergent boundaries newest magma deposits. This process would of course show a direct correlation between an oceanic plate's subducting angle and the distance to its opposing divergent boundary, and too, that boundary's proportional magmatic infill. When the mantle begins to subside; the greater that the ocean plate's expanse is, the greater the magma infill will be, which results in proportional compressional energies attained at the trench. The greater the plate is; the larger the tensional and compressional energies that will occur during the thermal cycle.
14<5.61 This prediction of course determines the ultimate speed that the plate will obtain. Wider plates = faster divergent boundaries metrics which = larger infills which = greater gravitational potential energies which = greater compressional plate energies which = greater subduction rates.
14<5.62 https://www.sciencedaily.com/releases/2010/07/100716125841.htm
14<5.64 The subducted section of ocean plate is now moving down deeper, the crust moving as the compression is released. As these thermal cycles repeat over tens of millions of years the subducted plate edge is stretched and slightly flattened by periodic extreme tension episodes that occur every five to ten thermal cycles, after which the particular ocean plate resumes the subduction that is otherwise almost continually driven by the compression in the crust. The periodic extreme tension that produce the stretch and flattening of the subducted plate section will pull the back-arc towards the trench during these rarer thermal expansion periods when the GPE in the crustal section is almost completely eliminated and the plate can thus be stretched between its two opposing ends. As was the case that resulted with the Mariana Trench being diametrically opposed to the Basin and Range Province.
14<5.65 When the subducted plate is pulled back its underside moves against the transitional boundary that it is in-bedded into, causing the subducting plate to move up and back under the overriding plate. This more extreme extension of the volcanic arc system towards the trench allows increased magma influx in the back arc fracture that keeps the island arc from regressing during the next compression period when the ocean plates will continue their more standard subduction again.
14<5.66 Those same periodic extreme tension periods that first broke the ocean plate away from the continent and then later periodically advance the island arcs towards the trench would undoubtedly re-fracture any large oceanic plates adjacent to where it is held in a convergent trench.
14<5.67 A remarkable research paper has been published that has changed the common understanding of convergent boundary dynamics while simultaneously supporting this model’s understanding of mantle dynamics and its ability to predict the research paper’s conclusions.
14<5.68 https://www.researchgate.net/publication/311446628_Metamorphic_record_of_catastrophic_pressure_drops_in_subduction_zones
December 2016, Nature Geoscience 10(1)
DOI:10.1038/ngeo2852Philippe Yamato, Université de Rennes
Jean-Pierre Brun, Université de Rennes 1
14<5.69 Abstract
“When deeply buried in subduction zones, rocks undergo mineral transformations that record the increase of pressure and temperature. The fact that high-pressure metamorphic parageneses are found at the Earth’s surface proves that rock burial is followed by exhumation. Here we use analysis of available data sets from high-pressure metamorphic rocks worldwide to show that the peak pressure is proportional to the subsequent decompression occurring during the initial stage of retrogression. We propose, using a simple mechanical analysis, that this linear relationship can be explained by the transition from burial-related compression to extension at the onset of exhumation. This major switch in orientation and magnitude of principal tectonic stresses leads to a catastrophic pressure drop prior to actual rock ascent. Therefore, peak pressures are not necessarily, as commonly believed, directly dependent on the maximum burial depth, but can also reflect a change of tectonic regime. Our results, which are in agreement with natural data, have significant implications for rock rheology, subduction zone seismicity, and the magnitudes of tectonic pressures sustained by rocks. Current views of subduction dynamics could be reconsidered in that perspective.”
14<5.610 What these researchers found was exactly what this model predicted; that a subducting plate that was loaded prior with GPE derived compression would be subjected to periodic tension as the oceanic plate was displaced during a typical mantle thermal pulse period when its subducted plate end was held fixed within the crust/mantle boundary while the connected seafloor portion was subjected to the shear forces of the mantle thermal pulse displacing the mantle.
14<5.612 The portion of the subducted plate that had been experiencing compression pressures higher than even the relative depth would impose was almost instantaneously put in tension and recorded mineral transformations that exhibit the preliminary increase of compression pressure and then sudden catastrophic pressure drop that occurred from the mantle thermal pulse prior to actual rock ascent to the surface.
14<5.613 The researchers state that these findings appear in many settings;
14<5.614 “However, other field examples clearly show that exhumation occurred in extension (regardless of P–T estimates), driven either by slab rollback (for example, Mediterranean15) or by extension of the overriding plate (for example, Norwegian Caledonides14,18 or Papua New Guinea25). It is remarkable that, whatever the tectonic setting, P–T data respect the same linear relation between peak pressure and pressure drop (Figs 1b and 3a), indicating that even where extensional exhumation is not obvious a transition from compression to extension probably occurred.”
-----------------------------------------------------------------------------------------------------------------
Page 15
Evidence of repeated episodes of
Slab advance, slab retreat and slab break-off
------------------------------------------------------------------------------------------------------------------------------------------------------------------------------------------------------------------
15<5.615 Is there evidence of these cycles in the geologic record? Evidence of repeated episodes of the model's mantle thermal pulses of 3-4 million year intervals? Episodes of slab advance and slab retreat, slab break-off that is then followed by subduction?
15<5.616 https://www.researchgate.net/publication/274265622_Repeated_slab_advance-retreat_of_the_Palaeo-Pacific_plate_underneath_SE_China
15<5.617 https://www.researchgate.net/figure/Cartoons-showing-a-repeated-slab-advance-retreat-model-a-ca-197-Ma-fl-at-slab_fig12_274265622
15<5.618 Repeated slab advance–retreat of the Palaeo-Pacific plate underneath SE China
Yao-Hui, Jiang; Guo-Chang, Wang; Liu, Zheng; Chun-Yu, Ni; Long, Qing; Zhang, Qiao
(2015): Repeated slab advance–retreat of the Palaeo-Pacific plate underneath SE China. figshare.
http://dx.doi.org/10.6084/m9.figshare.1328415Retrieved 04:37, Sep 08, 2015 (GMT)
15<5.619 "Integrating these observations, we propose a repeated slab-advance–retreat model for the late Mesozoic magmatic evolution of southeast China. Palaeo-Pacific plate subduction underneath southeast China initiated in the Late Triassic Rhaetian and reached southern Jiangxi by ca. 197 Ma, followed by slab rollback during 197–191 Ma and by slab break-off at ca. 189 Ma. Then slab advance was reestablished with the northwestward subduction approaching southern Hunan at ca. 178 Ma. From ca. 174 Ma, slab rollback reinitiated and gradually migrated from inland to the coastal area. This repeated slab-advance–retreat model is helpful to further understand the geodynamic mechanism of the late Mesozoic tectono-magmatism and related metallogenesis of southeast China."

----------------------------------------------------------------------------------------------------------------
Page 16
Prediction of
Double Benioff Zones
------------------------------------------------------------------------------------------------------------------------------------------------------------------------------------------------------------------
http://neic.usgs.gov/neis/eq_depot/2006/eq_061115_vcam/neic_vcam_c.html
16<5.620 The graph above and below are from USGS Earthquake Hazards Program. They show Magnitude 8.3 Kuril Islands earthquake Wednesday, November 15, 2006 at 11:14:17 UTC Seismicity Cross Section A to A at left and then below show slip on the fault and within the down going plate. Current research attributes the seismicity as a result of bending and extension as the plate is pulled into the mantle.
16<5.7 This model's thermo-kinetic displacement of the mantle would be a likely source of these double Benioff zones. Benioff zones were believed to be of a single layer of earthquake activity. Recent earthquake data has given a more accurate picture of Benioff zones and they show that almost all subduction zones have two parallel layers of earthquake activity. High pressure below 70 km (43.5 miles) should prohibit the release of strain that leads to earthquakes, but earthquakes at the Benioff zone can be as deep as about 700 km. I believe these are happening because of the currently occurring thermal expansion portion of the cycle.
16<5.71 As the mantle is slowly displaced it imparts shear force into the subducted plate. These forces produce tension at the subducted end of the plate where the displacing mantle is holding and applying tension to the embedded end, pulling it and reducing the deep compression forces that normally prevents the release of the deeper seismic energy. These acceleration/deceleration dynamic forces in the plate would simultaneously accommodate the currently observed subduction that is processing the present inventory of fossil gravitational potential energy that is in the planet's largest tectonic plates. In time, as the cycle of mantle displacement wanes, so would the deeper seismic activity, while the overall subduction may proportionally decrease or increase in response based on the amount of gravitational potential energy that will be realized as the mantle subsides and increasingly produces this driver of subduction.
-----------------------------------------------------------------------------------------------------------------
Page 17
Processing of accumulated
Sedimentary materials at convergent margins
------------------------------------------------------------------------------------------------------------------------------------------------------------------------------------------------------------------
17<5.8 There has been questions raised for the lack of sediments that should accumulate at the trenches over millennia, but the reciprocal movement of the trench opening allows the material to fall in deeper during expansion when the trench is pulled open wider. Then the material is compressed and subducted during contraction in a gravity fed conveyor that can process the volume efficiently. The trench opening width is partially determined by the crust's ductile properties in relation to the subducted plate angle. The tension applied is dependent on the distance to the divergent plate boundary. As the Pacific plate is gradually reduced in size in the future the shear forces that apply tension at the convergent trench will expand the divergent plate boundary in gradually decreasing widths while leaving the convergent trenches with ever decreasing applied tension during the expansion cycle.
17<5.81 I think those trenches are as wide as they will ever get. Some of the vertical movement in the overriding plate is the result of the repeated bending and straightening during the expansion-contraction cycles. This facilitates additional pulling traction between the subducted plate and the volcanic arc system that is overriding the subducted plate. It also transports the large volume of sedimentation materials and ocean water that are taken deep into the interior to be converted into magma and rock.
Images 1,2,3,4 and 5 used and modified by this author in the following section were furnished through and in no way endorsed by http://www.geomapapp.org using Global Multi-Resolution Topography (GMRT) Synthesis,
Ryan, W. B. F., S.M. Carbotte, J. Coplan, S. O'Hara, A. Melkonian, R. Arko, R.A. Weissel, V. Ferrini, A. Goodwillie, F. Nitsche, J. Bonczkowski, and R. Zemsky (2009), Global Multi-Resolution Topography (GMRT) synthesis data set, Geochem. Geophys. Geosyst., 10, Q03014, doi:10.1029/2008GC002332.Data doi: 10.1594/IEDA.0001000, through http://creativecommons.org/licenses/by-nc-sa/3.0/us/
-----------------------------------------------------------------------------------------------------------------
Page 18
A prediction of
Divergent boundary development
------------------------------------------------------------------------------------------------------------------------------------------------------------------------------------------------------------------
18<5.81 If this overall hypothesis is correct I would expect to see the latitudinal sections of oceanic crust that were historically the widest to be outpacing the narrower sections in ascendancy. Sometime earlier after the formation of the Pacific divergent plate boundary, but still a time when the axis was mostly aligned, these wider sections would begin to noticeably outpace the narrower sections with infill. The evidence in this would be the wider sections are retracted at the divergent boundary farther during the thermal expansion portion of the cycle than the narrower sections are because they have the greater portion of the differential of movement that a wider plate would have over its adjacent match.
18<6.0 This would allow more magma to fill the divergent boundary on the widest plate's side of the ridge axis. And in time as the thermal cycles repeat, that side of the axis would start to outpace the opposing latitudinal sections in the amount of magma infill. The ocean crust could adjust a little, but would eventually fracture in regular segments across the divergent axis. As tens of millions of years passed we would see large and ever growing fracture zones containing transform faults evenly distributed along the axis. The axis segments within the fracture zones would show in proportion the amounts of the differential movements each latitudinal section can attain when the boundary responds to the mantle displacement cycles.
18<6.1 Image 2. Such a pattern is visible in current relief maps of the Pacific Ocean floor. In the southern Pacific one can see the pattern in the western side of the ridge between the Challenger (A) and Eltani (B) fractures, it being the dominate half with the most allowable movement. It's inferior counterpart is an expanse of Antarctic plate that is fractured and faulted and is currently being extruded through the Strait of Magellan into what is now the separate Scotia Plate. The opposing and dominant seafloor subducts into the Kermadec (E) and Tonga (F) Trenches.
18<6.13 Thus, when either one of two opposing oceanic plates first fails and separates from its adjoining continent, it would undoubtedly precede its opposing plate section's eventual failure by a substantial period of time. This would allow that still attached section the lead time to establish and maintain a slowly increasing numerical advantage of additional ridge infill on that plate section's side of the ridge axis. This advantage is due to the additional differential of allowable movement that a still attached plate has over its opposing detached twin. These crescent sections of ridge that we now observe bear witness and give measure to that distant time period.
18<6.14 In this USGS image you can see the plate boundaries in their relative sizes and respective positions. Challenger (A) and Eltani (B) fractures are shown as are the Kermadec (E) and Tonga (F) Trenches.
18<6.21 And in doing so offset its axis in the opposite and inferior direction to the greater degree. This phenomenon is only relevant where one oceanic plate has a large advantage over the opposing plate, such as when the inferior plate is being reduced through subduction. And as time passes, inversely multiplying the small advantage the larger plate has.
18<6.22 This model would predict that these dynamics would eventually change from the initially superior side to the inferior side, and that many of the plate sections that now show evidence of past superior advantage will now in fact have current boundary axis metrics in the opposite dynamic.
18<6.23 For example; if the opposing overriding plate that is slowly consuming the inferior plate at a convergent boundary resembles something like that of the current arrangement of the N. American continent with its attached Atlantic oceanic crustal margin, this model would predict that the slowly increasing divergent boundary metric expanse on its Atlantic oceanic plate margin would produce a gradually increasing superior bias in counteraction to the Pacific divergent margins now comparably slower and decreasing metric rate. This would allow the divergent boundary plate remnant that now lies under the edge of the N. American western margin to be pulled by the combined metric expanse of the N. American continent and its adjoined oceanic plate section as the repeated mantle cycles incrementally displace the Pacific divergent boundary. Thus, we would see these various boundaries shift their spreading rates from one side of the boundary axis to the other as the adjoining continental/oceanic plate sections slowly increase or decrease their metric expanse in response to the changing plate dynamics that greatly influence the boundary axis metrics.
----------------------------------------------------------------------------------------------------------------
A model for
Atlantic divergent boundary dynamics
-----------------------------------------------------------------------------------------------------------------------------------------------------------------------------------------------------------------
19<6.3 The ridge area C is what I would expect a Mid-Ocean ridge (MOR) would look like around the time it fractured from the tension of thermal expansion. Notice the lack of fracture zones in this area. To the south is the large Chain fracture zone area (not shown) that has an enormous offset to the axis. And further to the south are some of the better examples of the ridge's offset segments.
19<6.31 Image 5. The opposing divergent sea floor and the continent's latitudinal similarity mirrors the ridge axis misalignment. The (MOR) section (B) between the Ascension Fracture Zone (A) to the north and the Bode Verde (C) to the south has a latitudinal distance imbalance from continental shelf to the ridge axis center of not more than 100 km out of the 2400 km of seafloor production on either side of the ridge, and taking the unknown continental shelf erosion into account, I assume they are almost equal in infill.
19<6.4 The section has a ridge axis misalignment (D) with the adjacent section to the north of 250 km +/-. Just to the south the next MOR section, which is bordered to the south by the Cardno Fracture Zone (E), has a ridge axis misalignment with the adjacent section to the north of 215 km +/- while the latitudinal distances to the continents are 2450 km +/- to the west and 2750 km +/- to the east, the imbalance is within the inaccuracy of the method of measurement. The MOR offset could be due to the east having more magma infill from more allowable movement but I think this has not yet developed into a measurable value compared to the overall ridge infill from uniform expansion.
19<6.41 As an example, the differential of allowable movement in the North Pacific Plate took much more plate width to achieve the gradual domination and curving of the ridge, while more importantly the opposing plate (Farallon) was being simultaneously reduced by subduction. Even though the South Atlantic MOR offset looks to be uneven it is in actuality very close considering it has been constructing sea floor for maybe 150 - 200 million years. So the Atlantic will be rather limited in this behavior compared to the Pacific until one side or the other of the Atlantic's oceanic crusts separate from its adjacent continent. When that occurs the oceanic plate still connected to a continent will have the most differential of allowable movement and gain the most infill on its side of the ridge axis. By the time this section eventually fractures and separates its counterpart will have been in subduction long enough that it cannot regain any advantage again, as the Pacific currently displays.
19<6.5 Why does the ridge have a 250 and 215 km offset? I suspect the offset in this and other ridge segments, including those that are nearly equal in latitudinal balance is due to the gradual increases and decreases that the mantle has sustained in its history of expansion and contraction cycles. The 215 km offset represents the total accumulation of ridge infill in proportion to that specific plate section's distance to its continental attachment point. The individual infills showing the magnetic field generator's specific heat flux and the resultant mantle circumference in comparison to past lower and probable higher thermal periods.
19<6.51 This is how the crust accommodates the ebb and flow of the thermal cycles where both oceanic and continental crust is radially extended and the sea floor is slightly raised from below by the mantle and the magma that is injected into the gradually opening mid-ocean ridge.
19<6.52 The lack of stress relieving mechanisms like convergent boundaries in the Atlantic oceanic plate sections requires them to accommodate the much greater compressive energies that are supplied by the adjacent continent's mass' as the mantle periodically and incrementally moves inward. These stresses will be greatly reduced when the oceanic plates eventually separate and can then process these gravitational potential energies into their own adjacent trenches.
19<6.6 The energy that the expansion has placed in the crust as raised mass will be slowly released as compression during contraction periods where energies would vary all along the MOR due to variations in ridge infill. At the time when the ancient continent fractured creating the Atlantic Ocean and Mid-Ocean Ridge the fracture offsets were probably non-existent, similar to area C in image 4. The expansion that followed was most likely caused from a large increase of core temperature. The planet had probably been in a long period of lower core energy and the sudden thermal increase is what initially broke the crust and restarted the gradual expansion of the mantle that was periodically interrupted by contraction episodes. These two directional movements of the ridge require latitudinal fractures to facilitate the increases and decreases in circumferences. As time passes the fractures will lengthen, smaller fractures may become dormant and fill with sediment giving over to larger and wider spaced fractures like those of the North Pacific's Mendocino south to the Marquesas. All of which are much wider spaced and longer in length.
19<6.61 Now we can see where a periodic pulse of hydraulic magma to overcome the almost unimaginable shear resistance/adhesion is really a possibility. When the Atlantic ridge opens during thermal expansion its allowable movement involves not just a 10 km thick oceanic crust like the Pacific but four massive continents, their rigid mass locking in that serpentine axis and preventing uneven movement. You can easily see why the North Atlantic only expanse 25 mm a year compared to the Pacific’s expansion of 80-120 mm a year. The weight of the North American and Eurasian plates is limiting the allowable movement by their massive weight and tremendous shear resistance and adhesion. Most of the movement is in the lighter ocean plates and is proportionate to their distance to the continents which is proportionally less than the Pacific. The Atlantic started with almost no allowable movement, so each infill was incrementally larger over time with the ocean plate having slightly more movement with each cycle than the last.
19<6.7 As time goes by the gravitational potential energy in the vertical movement will increasingly become stored in the oceanic crustal mass as potential lateral compressive energy to be released during the thermal contraction portion of the cycle, most of it in the heavier continents that will then be transferred to the oceanic plates. This is in contrast to the expansion period when the oceanic plate plays the central role through its degree of differential allowable movement, initiated by the outward displacement of the mantle, that accommodates more movement than the continents because the mantle will move in the direction of least resistance, slightly lifting the ocean plate in addition to the radial expansion that engages all the crustal plates. The compressive load energy over time will cause compaction of the oceanic plate material, this is what is responsible for the thickening of the oceanic plates and making them more rigid and stronger.
19<6.71 There was a time in the past when the East Pacific Rise had most of its eastern oceanic plate (Farallon) and with the Pacific Plate had a combined ridge expansion somewhere near the present combined total of the Atlantic and Pacific. As the Atlantic incrementally increased in allowable movement and potential compressive energy the Farallon decreased in same. And the Pacific having the wider longitudinal distance to the adjacent continent gained the most infill and off set its ridge to the Farallon side.
19<6.72 There are probably several reasons the Farallon was lost first and the Pacific will survive a bit longer; one is the East Pacific Ridge was closer to Eurasia on the Farallon side of the ridge. And also, this section was probably the first to separate from its adjoining continent which allowed the Pacific side to establish a dominate lead. But, most importantly, the Eurasian continent not only outweighs the North American continent but it is buttressed by the Pacific plate that is subducting against the Eurasian continental margin. Against all of this the N. A. continent is much easier to move. I think a hydraulic magma is needed to facilitate not only the thermal expansion movement of such mass, but also the overriding of the Pacific plate by the North American and Eurasian continental plates.
19<6.8 The Pacific plate was once connected to the Eurasian continental plate until shear derived tension of the ever growing oceanic plate overcame the structural integrity of the western end near where the continental plate is now overriding the oceanic plate's western edge. The outward expansion of the mantle created tension on the oceanic plate, as the mantle displaced outward it applied a broad shear force to the underside of the oceanic plate, causing the plate to break off and thus allow the continental connected section of seafloor to rise in counter action to the weight of the main section of the Eurasian plate. The two formerly joined sections could then function as a divergent plate boundary for the remainder of the expansion cycle. Millions of years later when the thermal cycle changed back to cooling and compression the fractured oceanic plate edge began its new role as a subducting plate that will produce in the following thermal expansion periods many ever growing trenches and island arc chains with their accompanying back arcs.
19<7.0 When the supercontinent Pangaea started to break up 170 - 200 million years ago there was probably a convergent plate boundary on both east and west continental shelves with the current Pacific divergent plate boundary occupying a position somewhere on the opposite side of the Earth. This arrangement had maintained a balance until the expansive land mass succumbed to the constant cycles of thermal expansion and contraction which broke the massive continent into the smaller land masses we recognize today. The event may have been instigated by a period of higher solar magnetic induction that produced a multitude of higher thermal cycles in the mantle and crust. When the North American and African tectonic plates parted, the separation of the plates created a basin that became the Atlantic Ocean and opened up fissures in the Earth's crust triggering volcanic eruptions that lasted for 600,000 years. The outflows of lava covered more than 9 million square kilometers (3.5 million square miles), an area roughly equal to the continental United States. Scientists call this area the Central Atlantic Magmatic Province (CAMP).
19<7.1 The 200 million year journey of the new world continents has produced the broad sea floor of the Atlantic Ocean and through the mechanical leverage of gravitational energy acting on continuous deposits of ridge material has propelled the divergent land masses over the Pacific plates now shrinking convergent boundaries. As the Atlantic widens, tension during thermal expansion will gradually increase until the long extended ocean crust has enough frictional drag from shear forces to cause the ocean crust near the east coast of the America’s to fracture. This fracture will become a new convergent plate boundary with the continent overriding the ocean crust. At that moment the new "West" Atlantic plate will no longer be pushing the heavy North American plate and it will unload its preloaded gravitationally supplied movement into the new convergent zone. If the continental crust is too massive and the thermal expansion is possibly of a higher degree the repeated thermal cycles could break and extend the land mass at its weakest point. If a large thick continental crust was repeatedly flexed as shear forces were applied from below, the continent would then succumbed and separated during the following continuous thermal expansion/contraction cycles.
-----------------------------------------------------------------------------------------------------------------
Page 20A model for
Continental breakup
------------------------------------------------------------------------------------------------------------------------------------------------------------------------------------------------------------------
20<7.12 Left; Map of middle Mississippi River valley in the central U.S. showing topography (purple at about 47 m up to orange at about 360 m elevation). White heavy lines bound the Reelfoot rift. New Madrid seismic zone seismicity shown by white and blue circles, are evidence that earthquakes threaten the Mississippi, Ohio, and Wabash River valleys of the Central United States. Several of the largest historical earthquakes to strike the continental United States occurred in the winter of 1811–1812 along the New Madrid seismic zone, which stretches from just west of Memphis, Tenn., into southern Illinois. Black box outlines Transect study area.
(Base topographic map courtesy of Robert Smalley, Univ. of Memphis.)
20<7.16 These fractures would have backfilled immediately with debris and been additionally fractured and disconnected from their base during the contraction cycle. These blocks will continue to loosen and fall in subsequent expansion-contraction cycles. This process would work the loosened crust and fill material continually down into the developing primary plate fractures, lowering the valley floor. The belief that it is now “undergoing east-west shortening” could be the expansion cycle is only in play in this fault zone during high thermal periods, loading up the debris and setting up the fault for series compressive slips over a period of hundreds or even tens of thousands of years. The 1811-1812 events were compressive slip events of the debris. Gravity acting on the raised plate is compressing the debris in the fault, driving the process of periodic movement of debris in the fault as the shear forces under the plate do not yet have the energy to tear the continent in half .
Image above courtesy USGS
20<7.17 As the continentally connected section of seafloor slowly lengthens the stresses applied to this fault will increase in proportion. Will the oceanic plate fracture in time to save the continent? Time will tell.
20<7.19 A team from Purdue and Northwestern universities analyzed the fault motion for eight years using global positioning system measurements and found that the fault system is moving at a rate of less than 0.2 millimeters per year and there is likely no motion.
20<7.20 The asthenosphere's visco-elastic material is at depths between 100 and 200 km (62 and 124 miles) below the surface, but perhaps extending as deep as 700 km (430 mi). The thickness, high viscosity and pressure of the asthenosphere are key to the mechanics of this mechanism. The asthenosphere’s viscosity is that of a rocky material under extreme pressure and temperature with movement, that according to the current standard model, is of a convective nature that operates in time scales of tens of millions to hundreds of millions of years. This ultra-slow rotational movement of mantle material known as convection cells is one of the mechanisms that the current standard model proposes as the source of tectonic plate movement. Because research from 3D seismic tomography images of the mantle's internal structure has not produced evidence of this process in the years since its proposal, some in the field have made additional modifications to the theory.
19<7.6 The lithosphere, of which the continental crust, 25-70 km (31 mi) thick, and the ocean crust, 7-10 km (6.2 mi) thick, form the upper layer, makes up the tectonic plates that extend up to 200 km (124 mi) deep. The lithosphere rests on the asthenosphere which is itself the upper portion of the mantle. I think the divergent plate boundary "tension fractures" (ocean ridge) are filled with hydraulic magma from this region where the lithosphere meets asthenosphere. A point referred to as the isotherm and related to the transition between brittle and viscous behavior. Sometimes called “low velocity zone” the asthenosphere is thought to be plastic in nature but in a manner of extreme viscosity. The location of the “Differential of allowable movement” with its variable shear resistance is this transitional boundary. Because the ocean crust is thinner than continental crust the hot asthenosphere can lie close to the ocean floor, reaching to within 2 kilometers of where the crust is formed at the mid-ocean ridges, supplying a steady source of magma to form the new ocean crust.
-----------------------------------------------------------------------------------------------------------------
Page 21
A model for the
Dynamic operation of the
Core-mantle thermal expansion mechanism
-----------------------------------------------------------------------------------------------------------------------------------------------------------------------------------------------------------------
21<7.7 The current standard model for plate tectonics has many unanswered technical inconsistencies that I believe this model can answer. We have already seen how well the thermal cycle fits the basic requirements of the observations, but how does it fit the deep interior of the Earth?
21<7.8 It is basically the same as the current standard model with the crust and upper portion of the mantle forming the cooler and more rigid outer layer of the Earth, aka the lithosphere. The asthenosphere is supporting this movable load and is substantially hotter and denser. So far no difference. In both models the crust and upper mantle are capable of the thermal transfer of heat through conduction. The lower mantle is considered thermodynamically more isolated. It is however ideally suited to the transfer of energy in the form of strain energy from its forced displacement outward, able to deform in radius repeatedly for hundreds of millions of years without expressing substantial structural change or weakness. It is analogous to the simple toy ball we had when we were kids, the Super Ball. If one had a ball with the mantle's scaled down dimensions and possessing a center of thermally, or for experimentation, hydraulically expandable material to simulate the outer cores thermal expansion, a simulated cycle could be observed.
21<7.9 The thickness of the mantle is instrumental to the mechanical leverage that the mantle's displacement imposes on the crust. It can multiply the very small but almost infinitely powerful expansionary energy that exists at the molecular scale during the thermal expansion of the outer core's molten iron, and convert it into much larger lateral kinetic movement that manifests in the crust at the surface. It is analogous to the mechanical advantage attained through the gear ratios of mechanical energy transfer systems.
8.0 The current standard model has over the years been modified as the inconsistencies became apparent. One is the speed of the convective material rising up through the mantle is at timescales of tens of millions to hundreds of millions of years. It is slow in the transport of heat content that is required at the lithosphere to produce thermal and kinetic evidence of either convection cells or mantle plumes.
21<8.I The various surface phenomena have specific identifiable time periods, the Hawaiian Island - Emperor Seamount Chain and flood basalt eruptions like the Deccan Traps and Siberian Traps for example, have periodicities that challenge a mantle plumes ability to maintain a simple causational link to timing and volume.
21<8.11 In this model the conductive energy transfer in the mantle is still occurring but the thermal expansion in the core produces an instantaneous mechanical transfer of strain energy to the mantle's outer boundary. The mantle is uniquely suited to this process, its density is the product of gravity acting on its mass weight of 4,080,000,000,000,000,000,000 tons in combination with the heat of the field generator that has an estimated temperature at the outer core-mantle boundary of 4400 C (7952 F). The electromagnetic processes of the core's thermal expansion/contraction and the compressive energy of gravity has in the last 4.5 billion years given the mantle a high coefficient of restitution. The untold number of expansion cycles has increased the density and arranged the composition of the materials in a manner similar to the same processes used to make highly resilient materials, that being extreme high pressure and temperatures. This has given the mantle the properties to resist additional compression, requiring the lower mantle to telegraph toward the surface additional expansive/contractive strain energy movement imposed by the thermal expansion/contraction of the outer cores 4400 C liquid iron as the solar magnetic field's variability forces temperature flux.
21<8.2 Excerpts below were furnished through and in no way endorsed by Allan F. Bower
http://solidmechanic.../Chapter4_3.htm
Applied Mechanics of solids by Allan F. Bower
Solutions to simple boundary and initial value problems
4.3 Spherically symmetric solution to quasi-static large strain elasticity problems
4.3.1 Summary of governing equations of finite elasticity in Cartesian components
This section is intended to illustrate the nature of solutions to elasticity problems with large shape changes.
4.3.2 Simplified equations for incompressible spherically symmetric solids
4.3.3 Pressurized hollow sphere made from an incompressible rubber

21<8.3 Please refer to Dr. Bower's http://solidmechanics.org/text/Chapter4_3/Chapter4_3.htm to completely appreciate his understanding of the mechanical dynamics involved.
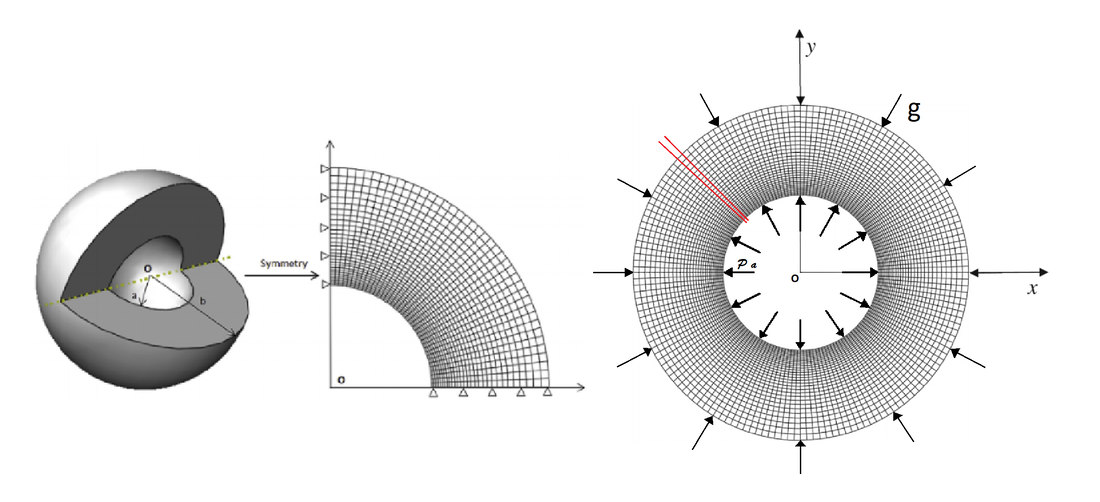
21<8.352 Eventually, some of these mantle tears, with their periodic and residual thermal content, will be located beneath weaker areas of the overriding crust as it is moved tectonically around the globe.
21<8.353 And periodically, some of these larger tears will align with the sutured areas at the margins between two tectonic plates, leading to the process of volcanic eruptions and discharges that precede the breaking up of a super continent similar to what is occurring at the Great African Rift Zone at this time. These larger thermal eruptions will eventually produce a nascent ocean basin.
21<8.36 These mantle tears may manifest as hot spot eruptive phenomena like an island chain or a Yellowstone volcanic zone. A larger tear could preheat the crust for several cycles and incrementally extend the overlying crustal area during the subsequent thermal pulse periods. These substantial mantle tears would be slowly reopening, and imposing their divergent dynamics into the overriding crust in such a manner as is currently happening within the Basin and Range Area in the S.W. United States.
21<8.37 And of coarse, if a massive solar magnetic period occurs there could be a Large Igneous Province discharge event as the voluminous amount of melted mantle material will force its way out into the lower pressure zone between the diverging crustal plates.
21<8.38 When the inverse square law is used to illustrate the outward displacement of the mantle the extreme surface tension at the crust/mantle boundary is easily understood. The resulting strain energy displacement would produce decompression melting of the surrounding boundary surface area materials, creating the hydraulic magma to displace the crust and fill the divergent plate boundaries.
Image above provided by Borb who has no connection to this author or this paper and was provided through Wikipedia Creative Commons.
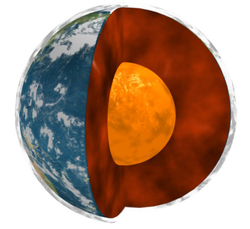
21<8.4 This description of the dynamic operation of the core-mantle thermal expansion mechanism shows a simple method of operation. Needing little more than gravity and the mass of the planetary components to produce, what are small by planetary scales, the outward strain energy movement that slightly extends the crust on a layer of hydraulic magma while slowly opening the divergent plate boundaries to the inflow, or more accurately the intrusion of magma into the mid-ocean ridge.
21<8.41 The outer core's thermal expansion that produces the strain energy, can press out the mantle faster than the outside radius of the mantle, due to its high viscosity, can expand in response. This causes tremendous tensile forces on the super viscose surface. These forces cause breaks or more like tears over the transitional boundary surface area of the mantle. This expansion driven tension release causes a reduction of pressure, allowing instantaneous melting of torn mantle surface and boundary area material. "Instantaneous" in this case is in scale to the phenomena, the surface area tearing would appear to us as ultra slow motion, comparable to the mid-ocean ridge spreading rates, as the large and small fractures are slowly pulled apart like cold taffy. A hydraulic magma is produced in tens of thousands of these tears and maybe millions of smaller ones. This fluid magmatic material is the hydraulic fluid that supports the tectonic plates. The energy released during this process keeps the fluid superheated.
21<8.42 If we could imagine the Earth having a transparent crust so as to observe the mantle in its natural state, we might wonder in more critical temperament how all of the hypothesized convective cells in the mantle could produce the observed movement in the divergent boundaries of the crust.
http://dx.doi.org/10...099.2013.835283
Australian Journal of Earth Sciences: An International Geoscience Journal of the Geological Society of Australia
Volume 60, Issue 6-7, 2013
D. L. Anderson
"Seismology, thermodynamics and classical physics—the physics associated with the names of Fourier, Debye, Born, Gr€uneisen, Kelvin, Rayleigh, Rutherford, Ramberg and Birch—show that ambient shallow mantle under large long-lived plates is hundreds of degrees hotter than in the passive upwellings that fuel the global spreading ridge system, that potential temperatures in mantle below about 200 km generally decrease with depth and that deep mantle low shear wave-speed features are broad, sluggish and dome-like rather than narrow and mantle-plume-like. The surface boundary layer of the mantle is more voluminous and potentially hotter than regions usually considered as sources for intraplate volcanoes."
21<8.51 This would suggest there is evidence that the crust/mantle boundary contains large volumes of magma that would be at play in providing a hydraulic medium between the mantle and crust, extending the crust while simultaneously extruding into the divergent boundaries as is currently being observed. The model proposes the magma is dependent on the strain energy that is simultaneously released by the mantle during its displacement.
"that potential temperatures in mantle below about 200 km generally decrease with depth"
21<8.52 That observation runs counter to the the standard model and the generally accepted belief that temperatures are lower nearer to the surface and increase with depth. A source and its accompanying mechanisms would be needed to explain this crust-mantle boundary temperature anomaly.
21<8.6 Referring back to that paper regarding the mantle's oscillations;
21<8.61 https://www.academia.edu/38174615/Mantle_thermal_pulses_below_the_Mid_Atlantic_Ridge_and_temporal_variations_in_the_formation_of_oceanic_lithosphere
Mantle thermal pulses below the Mid-Atlantic Ridge and temporal variations in the formation of oceanic lithosphere
Enrico Bonatti*†‡, Marco Ligi*, Daniele Brunelli*†, Anna Cipriani‡, Paola Fabretti*, Valentina Ferrante*†, Luca Gasperini* & Luisa Ottolini§
* Istituto di Scienze Marine, Geologia Marina, CNR, Via Gobetti 101, 40129, Bologna, Italy
† Dipartimento di Scienze della Terra, Universita` “La Sapienza”, Piazzale Aldo Moro 5, 00187, Rome, Italy
‡ Department of Earth and Environmental Sciences, Lamont Doherty Earth Observatory, Columbia University, Palisades, New York 10964, USA
§ Istituto di Geoscienze e Georisorse, Sezione di Pavia, CNR, Via Ferrata 1, 27100, Pavia, Italy
21<8.62 "We calibrated our spinel and opx Cr# data in terms of degrees of melting assuming as a source a fertile, pyrolite-like peridotite13,15. Degrees of melting calculated from Ti/Zr of cpx and from Cr# of opx and spinel correlate. They range along the VLS from 5.7% to 14.3%, with oscillations where the degree of melting appears to increase gradually and then decreases rapidly. These short-wavelength oscillations are superimposed on a long-term (.15Myr) trend of increasing degree of melting with time, estimated on a regression line to range from 7 ^ 1.5% about 19Myr ago, to 13 ^ 2% about 4Myr ago."
21<8.7 You can see in that description above the strain energy response of the mantle's outer surface to the core's outward displacement. As the viscosity resisted the mantle's surface tension, the outward expansion caused the mantle's surface to be shredded and torn and thus melted. Then when the outer core's thermal expansion eventually paused the mantle's "melting" did also as the temperature "decreased rapidly." Eventually the Earth's magnetic field strength increased further still and supplied another pulse of strain energy, and through that, the further melting of the crust/mantle boundary during another period of slowly increasing mantle melting followed by another rapid decrease as the strain energy waned once more.
21<8.71 The timing of these oscillations fit the surface observations remarkably well.
21<8.8 The Yellowstone Hotspot’s intermittent progress eastward across the N.W. U.S. displays the pause that allows the caldera to form. This stationary period coincides to when the mantle is incrementally displacing outward as magma is filling the slowly opening divergent boundaries around the world. This new ridge material will in turn provide the leverage to produce the westward advancement of the N. American plate that simulates the Yellowstone hotspot’s movement east during the mantle’s subsequent return towards the core.
21<8.81 The timing matches the paper's estimates;
"The degree of melting of the mantle that is upwelling below the ridge, estimated from the chemistry of the exposed mantle rocks, as well as crustal thickness inferred from gravity measurements, show oscillations of ,3–4 Myr superimposed on a longer-term steady increase with time. The time lag between oscillations of mantle melting and crustal thickness indicates that the solid mantle is upwelling at an average rate of ,25mmyr"
Image provided by Kelvin Case at English Wikipedia, who has no connection to this author or this work.
21<8.9 As you can see the fit is remarkable, this model matches not only the overall timing but the duration of the cycle segments of pause and then movement to the next hot spot location. Add to this the model's ability to furnish the energy to move the continental mass over the remains of the Farallon and the hot spot complex itself, makes this a very accurate prediction of observation.
Image above courtesy of USGS
21<8.91 The current explanation for the Hawaiian-Emperor Seamount Chain is that they are the result of a hot mantle plume from the core/mantle boundary or are the result of a lithospheric extension that allows the passive rising of melt from a more shallow depth.
21<8.92 The continuous plate movement of the standard model puts the responsibility of this island chain's periodicity of individual island building while progressing laterally on a continually moving ocean plate squarely on the magma source and the requirement that it must have the unique ability to produce the magma in pulses so as to build islands separated by time and distance. The fact that the standard model does not offer a satisfactory means to move the plates in the first place only compounds the model’s problems regarding this phenomenon.
21<8.93 The asthenosphere's visco-elastic material is at depths between 100 and 200 km (62 and 124 miles) below the surface, but perhaps extending as deep as 700 km (430 mi). The thickness, high viscosity and pressure of the asthenosphere are key to the mechanics of this mechanism. The asthenosphere’s viscosity is that of a rocky material under extreme pressure and temperature with movement, that according to the current standard model, is of a convective nature that operates in time scales of tens of millions to hundreds of millions of years. This ultra-slow rotational movement of mantle material known as convection cells is one of the mechanisms that the current standard model proposes as the source of tectonic plate movement. Because research from 3D seismic tomography images of the mantle's internal structure has not produced evidence of this process in the years since its proposal, some in the field have made additional modifications to the theory.
21<8.94 Mantle Plume Theory was suggested shortly after plate tectonics acceptance to accommodate the phenomenon of the Hawaiian Island - Emperor Seamount Chain. It was further developed in the 1990's to maintain convection as the central energy source for massive scale plate movement, explaining that the dividing of continents such as North America and Europe, required large scale plumes to rise from greater depths, possibly the outer core, with smaller plumes explaining smaller phenomenon. As inconsistencies developed modifications were proposed that include; mantle wind, Euler pole jerks, mantle roll, magma tunnels, hidden plate boundaries, lithosphere drift, super plumes, lateral flow, group motions of "hot spots", plume head decapitation.
21<8.95 https://www.earthmagazine.org/article/question-mantle-plumes
21<8.96 "“These methods reveal the seismic structure of the mantle,” says Gillian Foulger, a geophysicist at Durham University in England, and a longtime collaborator of Anderson’s. They “do not reveal the geological structure. The two are not the same.”. . . . .
21<8.97 . . . “Plumes have been proposed to come from almost any depth, to rise vertically or tilt, to flow for long distances laterally, to have narrow or broad conduits, to have no plume head, one head, or multiple heads, to produce steady or variable flow, to be long- or short-lived, to speed up or slow down, to have a source that is either depleted, enriched, or both, and to have either high or low [ratios of helium-3 to helium-4],” Foulger wrote in Geoscientist in May 2003.
21<8.98 She wasn’t alone in her thinking that the hypothesis was becoming too broad. In a letter to the editor of Geoscientist that year, Northwestern University seismologist Seth Stein wrote: “I wouldn’t blame anyone for the state of thinking … about hot spots and mantle plumes except ourselves. As in Pogo’s dictum, ‘We have met the enemy and they are us.'”
21<8.99 Stein added that in the “absence of any other clear model,” the geoscience community had accepted “very vague ideas about plumes” and allowed them to become the “null hypothesis” for anomalous volcanism. He noted that although the hypothesis initially entailed rigorous criteria — for example, the presence of a low-velocity zone, age-progressive island chains and near-fixity — the science had progressed to the point where “plumes don’t have to meet any particular test.”
21<8.991 “Hence the hypothesis now always works with appropriate site-specific modifications, but increasingly doesn’t tell us anything or predict anything, especially about structures formed in the past,” he wrote. “It does, however, make it harder to offer nonplume explanations.”"
21<9.0 The Plume model appears to be a rather incomplete and complicated explanation that requires a tenuous expectation of the source’s ability to maintain such consistency over what likely extends beyond the farthest currently observed position of the Hawaiian–Emperor seamount chain system, considering the oldest seamount dates to where the chain is now being consumed into the Kuril–Kamchatka Trench 5,800 kilometers from the source’s current location suggests it could be much older still.
21<9.01 As was mentioned at the introduction of this model where the many inconsistencies of the idea that the mantle is possibly convecting from deep within the Earth and engaging the underside of the tectonic plates were briefly mentioned;
21<8.902 "But very recent research observations have made this basal drag mechanism most likely non-existent. There has always existed a non sequitur in this idea. Holmes' convection mechanism came into full acceptance when plate tectonics coalesced together with the mantle plume theory proposed by J. Tuzo Wilson in 1963 and further developed by W. Jason Morgan in 1971. The fundamental problem has been mostly ignored up till now but a recent research paper has delivered a seemingly insurmountable observation that nullifies the idea that the mantle is flowing horizontally and tugging the overriding tectonic plate along with it."
21<8.903 "In short, the research shows that Easter Island is slightly younger (2.5 Ma) than the section of tectonic plate it sits on that is not much older than Pliocene, 3–4.8 million years old. But certain zircon crystals on the Island have been found to be as old as 165 million years."
21<8.904 https://phys.org/news/2024-10-easter-island-volcanic-history-earth.amp
21<8.905 "The classical "conveyor belt theory" was already difficult to reconcile with the observation that mantle plumes stay in place while everything around them continues to move. Van Hinsbergen says, "People explained this by saying that plumes rise so fast that they are not affected by a mantle that was moving with the plates. And that new plume material is constantly being supplied under the plate to form new volcanoes."
21<8.906 "But in that case, old bits of the plume, with the old zircons, should have been carried off by those mantle currents, away from the location of Easter Island, and could not now be there at the surface."
21<8.907 "From that, we draw the conclusion that those ancient minerals could have been preserved only if the mantle surrounding the plume is basically as stationary as the plume itself,""
21<8.908 https://d197for5662m48.cloudfront.net/documents/publicationstatus/179885/preprint_pdf/eb9bc66de0f79ec5d2400024844103e0.pdf
Zircon xenocrysts from Easter Island (Rapa Nui) reveal hotspot activity since the middle Jurassic
Yamirka Rojas-Agramonte, Natalia Pardo, Douwe J.J. van Hinsbergen, Christian Winter, Mar´ıa Paula Marroqu´ın-G´omez, Shoujie Liu, Axel Gerdes, Richard Albert, Shitou Wu, and Antonio Garcia-Casco
November 29, 2023
21<8.909 "If our hypothesis is valid, the geodynamic implications are far reaching. It would suggest that not only the Galápagos and Easter hotspots are nearly mantle-stationary, but also that the asthenosphere in which the underlying plume is contained did not appreciably move for the last 165 Ma. Because if the mantle would convected and moved laterally with a plume fed from below continuously piercing ‘fresh’ asthenosphere, the older melt relics and zircon cargo would have convected away. . . ."
21<8.910 ". . . . Our findings show that asthenospheric mantle-derived xenocryst zircon cargo, such as that recently reported from Galapagos and Hawai’i, may not be an exception. The "ghost" of prolonged hotspot activity described here suggests that the Easter hotspot as well as the sublithospheric mantle in which it is located remained stationary for some 165 Ma, which, if true, has major implication for our understanding of mantle convection Even though we consider our results preliminary, we conclude that zircon geochronology combine with elemental (REE) and isotopic studies of young oceanic islands may offer a novel source of information that could upset our thinking of global-scale geodynamics."
21<8.911 Every indication in the research above and the many others that the authors reference indicated that the reigning theory of the driving force of plate tectonics, where the mantle is moving horizontally under the tectonic plates and is tugging them along with a basil drag coupling, is now demonstrably untrue.
-----------------------------------------------------------------------------------------------------------------
Page 22
Model of
Hawaiian-Emperor Seamount Chain
-----------------------------------------------------------------------------------------------------------------------------------------------------------------------------------------------------------------
22<9.1 I have looked for the “tree rings” of the magnetic field generator’s thermal cycle, a solid readable record of the mantle thermal cycles of the planet, the Yellowstone is a very convincing example and I think I found another. I think the Hawaiian Island chain and its undersea ancestors of the Emperor Seamount Chain are the record I’m looking for. Let’s take a look at the evidence.
Image above was acquired from GeoMapApp at http://www.geomapapp.org using Ryan, W. B. F., et al. (2009), Global Multi-Resolution Topography synthesis, Geochem. Geophys. Geosyst., 10, Q03014, doi:10.1029/2008GC002332.
22<9.14 The seamounts and the hotspots that make them are a thermal expansion dependent mechanism, or more specifically, what is simply a large deep tear in the mantle's surface from past historic crust/mantle boundary hyperthermal events. To illustrate this idea, we need to imagine how the mantle would respond to a outward force from within as the core's magnetic field generating components thermally expanded, and further how the mantle would respond in kind as its entire outer surface is being subjected to immense multi vector lateral tensions. We would see that these higher tractions would manifest in locations on the mantle's surface far away from continents and their adjacent boundaries where higher mantle thermal process' already manifest as subduction and volcanic phenomena.
22<9.14.1 The broad expanse of a cooler mantle surface located centrally under a broad ocean basin like that of the Pacific's would be the most vulnerable to such deformations. And as would be expected, the resultant, and increasing, strain energy would eventually locate the many weakness' that exist anywhere on the mantle's highly viscous surface that could not resist the immense strain tensions applied to them. The center of these ocean basins may be the incubator for these long lasting residual thermal mechanisms that may eventually be overran by a continent and produce phenomena like the Yellowstone complex and Basin and Range like extensional environs. Undoubtedly, the cooler mantle's surface under the Pacific's would not stretch in the familiar somewhat even manner like a thin balloon would. Its hot, dense and viscous rock would instead mostly resist the surface tension until a weakness is exposed and suddenly torn. This sudden rapid release of the immense tension of the affected areas produces the sudden lower pressures that allows the surrounding materials to melt.
22<9.15 These phenomena would occur all over the mantle's outer surface, in a wide range of sizes, with some large enough to be what we would observe to be as a hotspot, like the one we now observe under the Hawaiian Islands. These would ideally be what would weaken the crust above it and exploit the divergent lateral forces produced by the mantle thermal cycles to create a nascent triple junction and begin the divergent boundary process where none had been prior. This would of course follow the processes laid out here already where the thermal cycle would subside and the divergent boundary would compress the recent infill to produce compression in the crust. While back at the island chain, once again, the Hotspot/Mantle-tear would reform into the now cooler thermal plastic mantle rock, and remain as such, until the next solar magnetic increase that begins the process' that would again exploit and reactivate that still thermally weakened region, initiating localized strain energy once more and producing again the passive melt that propagates through the weakened crust to begin another nascent island.
22<9.16 This Hotspot/Mantle-tear would stay located in the mantle where its residual thermal footprint was born, even though the oceanic plate anomaly above is moving on with the migrating plate, but we could imagine the mantle having uneven directional surface tractions from various other directions that would pull on the tear more from one direction than another during the following cycles and would thus over time experience increased tractions from both the crust and mantle from one particular direction than the others, leading to the Hotspot/Mantle-tear migration overtime. The Hotspot/Mantle-tear began and would of coarse continue to produce the molten magma during each following mantle thermal cycles.
22<9.17 So, we've gone from an obscure point on the mantle underneath the middle of an oceanic plate, to where simple mantle dynamics leads us to how a chain of island/seamounts are established and simple surface tension variation dynamics on the mantle can tug a Hotspot/Mantle-tear in an appreciable direction and distance over time. And, if these energies were to ever rise to the appropriate levels, this island building thermal anomaly could become a much larger expression of divergent forces and weaken the overriding crust enough to produce a triple junction that may someday develop into a divergent boundary.
22<9.171
http://www.mantleplumes.org/Hawaii2.html
Speculations on tectonic origin of the Hawaii hotspot
Ian O. Norton
ExxonMobil Upstream Research, Houston, Texas, USA
22<9.18 "Figures step though time showing areas of sea floor appearing as they are created" . . . . "with the area north and west of the trench blanked out. This is done to emphasize that the sea floor we are dealing with was a long way from any continental margin when it formed"
22<9.181 "Anomalous topographic features in the area were created in several ways: Shatsky Rise by excess ridge axis-type volcanism at a triple junction (Sager et al., 1999; Mahoney et al., 2005); Hess Rise by excess volcanism at a ridge-transform intersection perhaps associated with a spreading direction change (Figure 6); Chinook Trough by ridge reorganization also associated with a spreading direction change (Figure 9); Emperor Trough as a fracture zone (Figure 8) and Meiji Seamount at a spreading ridge associated with both a spreading direction change and perhaps at the ridge left behind by a ridge jump"
22<9.182 "Geochemical evidence presented by Keller et al. (2000), particularly strontium, lead and neodymium ratios, are also consistent with formation of Meiji and Detroit seamounts close to a ridge axis. I suggest here that Meiji formed at the Pacific – Kula/Izanagi ridge axis as a result of excess volcanism associated with the 30° change in spreading direction from 84 to 71 Ma shown in Figures 9 and 10. As inferred in Figure 10, Meiji may actually have formed at an abandoned spreading center left behind as north-directed spreading became established."
22<9.183 "Meiji is the oldest preserved seamount in the Hawaii-Emperor seamount chain. Whether there were older seamounts in the chain that have been subducted is, of course, not known. However, if the inference from gravity data, isotopic data and the plate boundary scenario presented above that Meiji formed at a ridge axis is correct, it is likely that Meiji is the oldest seamount in the chain. If this is so, and it formed by processes related to sea floor spreading, an important implication is that the Hawaii hotspot also formed by processes initiated at the (abandoned?) spreading ridge. This means that the hotspot formed as a result of spreading processes and plate tectonic motion. It would, of necessity, have a shallow (upper mantle) origin. This is different to the usual model for a hotspot as being derived from the mantle or core remote from the influences of plate tectonic processes and thus superimposed on plate processes, with little interaction between the two. The shallow model is along the lines of the "plate model" for the Earth proposed by Anderson (2005) and the "alternative Earth" of Hamilton (2003)."
22<9.184 As you can see by the descriptions above, this model's straight forward and simple idea is a best fit over any other current model.
22<9.19 These phenomena are pressurized with hydraulic magma from the crust/mantle boundary area during the thermal expansion of the mantle and are slightly raised causing the crust to be stretched and thinned, eventually cracked when positioned over the hot spot exposing the crust to high levels of superheated magma.
22<9.2 The length of the thermal event determines if the stay over the hot spot is long enough to build an individual island or just a short period that produces a small seamount on the ocean floor. A series of short cooling periods interrupting an overall thermal period would build a chain of superimposed volcanoes as the crust incrementally shifted and then continued lava discharge. Eventually the core begins cooling, and as the high heat content of the crust/mantle boundary moves into the crust and ocean, the fissures are slowly closed causing the volcanoes to become dormant. The crust then moves towards the core increasing the compression against the most recent ridge infill and continues its journey towards the direction of least resistance.
22<9.23 Thus the spacing indicates that the cycles were mostly dominated by small mantle pulses, especially for the area following the bend. When the mantle was in outward displacement the seamount building periods in these areas appear to have been shorter and/or of lower energy. It would seem that there were simply a series of cycles containing very low energy and/or short periods of outward displacement of the mantle. An important point to note is even though the sizes of the mounts differ greatly between (B) and (C) the actual center to center distances between either group appear to be very similar and would lead me to propose that the speed of the plate's movement during each of these periods was rather similar, area (B) is a very long period of lower energy "mantle thermal pulses", and look's to have actually increased overall from the oldest to the newest.
Part 2
Page 23 A mechanism for producing hydraulic magma at the crust/mantle boundary
Page 24 Hotspot formation and large eruptive discharge events
Page 25 Mechanism for high speed continental movement
Page 26 Mechanism for Mountain formation
Page 27 Model for the creation, storage and distribution of: Compression and Gravitational Potential Energy within the crust
Page 28 Model for globally simultaneous boundary deformation with subsequent and significant carbon isotope excursions and mass extinctions (Evolutionary Mechanism)
Page 29 Correlation of extensional and compressional episodes to Miocene, Pliocene and Pleistocene temperature record.
Page 30 A model for Mountain ranges on Continental interiors
Page 31 A model for plate dynamics of oceanic slab break-off and relocation Page 32 Evidence of Periodic Extension/compression Cycles in Oceanic Lithosphere
Page 33 A few images of crustal compression at work
Page 34 Summery
-------------------------------------------------------------------------------------------------------------------------------------------------------
Part 2
Page 23
A mechanism for producing
Hydraulic magma at the
Crust/mantle boundary
-----------------------------------------------------------------------------------------------------------------------------------------------------------------------------------------------------------------
23<9.1 As the Earth warms during a long term solar magnetic increase, the periodic expansion of the outer core for a period lasting 1 million years or more would produce at the slowly expanding surface of the mantle, what looks to be as the melting away of the surface. The volume of hydraulic magma stays within the observed parameters of;
- the solar magnetic energy strength.
- the core/outer core thermal flux
- the degree of mantle displacement
- and the mid ocean ridge infill
23<9.11 The resulting magmatic fluid that is produced at the many locations where the mantle surface becomes torn from the strain tension, enables the frictional movement that is applied to the tectonic plates by the displacing mantle. The eventual cooling that periodically divides the thermal cycle marks the mantle's reclamation of the magma sources through, again, temperature and pressure, reforming the mantle material into the thermoplastic rock that supports the outer shell of the planet.
23<9.2
https://www.nsf.gov/news/news_summ.jsp?cntn_id=127315
March 20, 2013
Scientists Discover Layer of Liquefied Molten Rock in Earth's Mantle
Hidden magma layer could play role in shaping the geologic face of our planet
23<9.21 "Scientists have discovered a layer of liquified molten rock in Earth's mantle that may be responsible for the sliding motions of the planet's massive tectonic plates." . . . ."The finding may carry far-reaching implications, from understanding basic geologic functions of the planet to new insights into volcanism and earthquakes." . . . ."The scientists discovered the magma layer at the Middle America trench off Nicaragua's shores."
23<9.22 "the scientists imaged a 25-kilometer- (15.5-mile-) thick layer of partially melted mantle rock below the edge of the Cocos plate where it moves beneath Central America."
23<9.23 "For decades scientists have debated the forces that allow the planet's tectonic plates to slide across the Earth's mantle."
23<9.24 ""Our data tell us that water can't accommodate the features we are seeing," said Naif. "The information from the new images confirms the idea that there needs to be some amount of melt in the upper mantle. That's what's creating this ductile behavior for plates to slide.""
23<9.25 ""One of the longer-term implications of our results is that we are going to understand more about the plate boundary, which could lead to a better understanding of earthquakes," said Key.""
23<9.26 "The researchers are now trying to find the source that supplies the magma in the newly discovered layer."
23<9.27 This model's simple mantle displacement mechanism provides that source of boundary area mantle melt.
23<9.3
http://geoprisms.org/newsletters/imaging-the-nicaragua-subduction-zone-with-marine-electromagnetic-methods/
Imaging the Nicaragua Subduction Zone with Marine Electromagnetic Methods
Samer Naif1, Kerry Key1, Steven Constable1, Rob L. Evans2
1SCRIPPS Institution of Oceanography, 2Woods Hole Oceanographic Institute
Modified from Naif, S., Key, K., Constable, S., Evans, R.L., 2013. Melt-rich channel observed at the lithosphere-asthenosphere boundary. Nature 495, 356-359.
23<9.31 "There are three competing hypotheses often invoked to explain the oceanic LAB that is prominent in seismic and EM observations: 1) a thermal boundary, 2) a hydration boundary, 3) a partial melt boundary (Fischer et al., 2010). From the existing laboratory studies of the electrical conductivity of expected mantle phases, the signature of our observed channel is too conductive to be explained with either temperature or hydrated olivine alone. Such high conductivity measurements require an interconnected network of 1-2% hydrated basaltic melt. Alternatively, a recent study suggests that 0.3-0.5% of highly enriched incipient melt is a more plausible interpretation (Sifré et al., 2014)."
23<9.4 This mechanism allows the mantle to transform the core's thermal expansion increase into strain energy displacement at the core-mantle boundary, and then convert a large part of it from strain displacement back to thermal energy at the surface of the asthenosphere. It is a mechanism that can quickly transport the core's thermal derived expansion as strain energy movement through the mantle to the crust. This allows the solar magnetic field's variability to influence, even impose short and long time scale thermal forcing into the Earth's crust, ocean and biosphere. The timing of the short and long cycle periods can be compared to the periodicity of Ice Age and glacial-interglacial events, where larger cycle periods are divided by smaller two way transient movements of temperature of which they are most likely connected. The upper mantle may work as a heat sink, moderating the heat cycle. The phenomenon is supplying large volumes of magma that “float” the plates and furnish divergent plate boundaries with magmatic building material.
-----------------------------------------------------------------------------------------------------------------
Page 24
Hot spot formation and
Large eruptive discharge events
24<9.5 There are a number of historic basaltic eruptive events that I believe are related to large displacement caused mantle-tears in the mantle's surface; these are deep expansion fractures from past high thermal events. Siberian Traps of 250 million years ago, the Ontong Java Plateau at 125–120 million years ago and the Deccan Traps at the end of the cretaceous period 60 - 68 million years ago. And I think they are related to the various current hot spot phenomenon that include the Hawaiian Islands, the Yellowstone complex, Iceland, Réunion, and Galápagos to name a few.
24<9.51 But first Iceland, if this is a hotspot has it stayed aligned with the Atlantic spreading center since the continents first separated? In the new model the mid-ocean ridge resulted from the lack of a differential of allowable movement in the oversized continental tectonic plate, resulting in it succumbing to the strain energy displacement of the upper mantle from thermal expansion of the magnetic field generating components. These old sutured seams joining the assembled cratons together will eventually yield to the persistent cycles of mantle displacement.
24<9.52 But, could a hotspot like Yellowstone have assisted the process? Could the one under Iceland have been there from the beginning when the continents first separated? Was it because the preexisting sutured seam that first activated and created the Mid-Atlantic Ridge was directly over where the mantle produced a mantle hotspot like the Hawaiian-Emperor chain? Or did it arrive there by way of geologic processes similar to Yellowstone's current situation?
24<9.53 Image above used and modified by this author was furnished through and in no way endorsed by://www.geomapapp.org using Global Multi-Resolution Topography (GMRT) Synthesis,
Ryan, W. B. F., S.M. Carbotte, J. Coplan, S. O'Hara, A. Melkonian, R. Arko, R.A. Weissel, V. Ferrini, A. Goodwillie, F. Nitsche, J. Bonczkowski, and R. Zemsky (2009), Global Multi-Resolution Topography (GMRT) synthesis data set, Geochem. Geophys. Geosyst., 10, Q03014, doi:10.1029/2008GC002332.Data doi: 10.1594/IEDA.0001000, through http://creativecommons.org/licenses/by-nc-sa/3.0/us/
24<9.6 Yellowstone now lies under western North America. Yellowstone's extinct historic surface anomalies trailing across Oregon, Idaho and Wyoming. Mantle plume theory proponents claim these hotspots are the result of a plume, while large scale convection currents are believed to be causing the oceanic plates to separate at the divergent boundaries. But does that scenario really work in the case of the Atlantic? The North American and African tectonic plates rifted apart 200 million years ago, the resulting basaltic lava lasted for 600,000 years and covered more than 9 million square kilometers (3.5 million square miles).
24<9.7 This model can accommodate the mantle plume hypothesis and convection cells, but the plume hypothesis' complexities muddy the waters of the current standard model and would do the same with this hypothesis. And under closer examination it would seem difficult for convective currents to exist and maintain continuity in a mantle that was expanding and contracting in 3-4 million year periodicities. Increased complexity is always moderated by reduced probability. Occam's Razor favors the least number of mechanisms to accomplish the task and this hypothesis gains nothing through adding unneeded processes. The model’s simple transfer of energy through electro-thermo-kinetic relay produces causative mechanisms for any and all current phenomenon that mantle plume and even convection cell hypotheses tenuously attempt to explain.
24<9.71 Mid-Ocean ridges are the result of a continental break-up that forms a new oceanic crust spreading center and a future ocean basin such as with the Atlantic. The model contends that during larger solar magnetic induction periods the mantle boundary surface will be stressed and acquire tension to an increased proportion. Due to the mantle's thermoplastic nature it will tear at thermally higher, and by that weaker boundary surface area locations. These will be symmetrically located across the mantle boundary area surface in proportion to the mantle's coefficient of restitution. These areas are where mantle heat and friction during the displacement are more likely to be concentrated, under large continental structures. Additionally the friction of the differential of allowable movement in the slowly spreading, and by that stretching continental crust would produce additional divergent mechanical force on the mantle's boundary surface, further adding the formation of these large magma and thermal reservoirs. This would provide a continuous process for continental break-up and seafloor generation to the already hypothesized cycles currently studied in this paper.
24<9.8 This model proposes the ancient hyper thermal extinction events are simply in line with what would be expected if solar magnetic energies were high enough to produce large expansion and production of magmatic fluid that exceeded the differential of allowable movement of the crust, resulting in the expansion fracturing of the crust and lava discharges. The solar magnetic energy levels that would produce large discharges would require the mantle to expand and fracture its surface in large tears producing large volumes of hydraulic magma. The surface tension in the crust will build to critical levels until the crust fractures at its weakest point from the extreme tension. The crustal fracture would likely occur directly over a large mantle tear where the most heat and pressure would be present to weaken the crust.
24>9.81 The larger mantle tears take longer to re-close due to the mantle's high viscosity, and remain filled with residual magma for protracted time periods that are in proportion to the energy that created the hyperthermal event. These larger thermal expansion periods cause the mantle surface to expose the overlying crust to extreme levels of heat and pressure. As the mantle radially expands beyond the crust's rate of allowable/or differential of movement the crust will fracture at its weakest point, allowing ejecting lava out of the fracture for the duration of the solar magnetic field's high energy period. These are the hyperthermal events that include the historic Siberian Traps of 250 million years ago, the Ontong Java Plateau at 125–120 million years ago and the Deccan Traps at the end of the cretaceous period 60 - 68 million years ago.
24<9.9 I also believe the fractures in the crust close up proportional to the thermal contraction and in that way can be controlled from a deep fissure that uses the thermal contraction of the mantle to control high pressure magma. These are reservoirs of magma that can transfer a large degree of concentrated heat energy into the crust.
24<9.91 The Hawaiian and other island chains along with Yellowstone are different from ridges due to a smaller heat source in comparison to the larger scale of the volcanic activity at the mid-ocean ridge spreading centers like that of the Atlantic that are aligned to a larger magma source that allows a more passive discharge. In the most simple analogy the smaller island anomalies are high pressure leaks, due to a particular structural feature that results when a mantle tear is formed. When a thick walled spherical body of highly resilient material fails from extreme surface tension the surface will be torn in multiple places where the edges of the failures are pulled back and up as the localized tension is released. These are opened and activated only during the thermal expansion of the mantle and are slightly raised causing the crust to be stretched, thinned and cracked when positioned over these structures, exposing the crust to high levels of thermal energy. When the mantle eventually subsides causing the hotspot/mantle-tears to reform once again into the thermal plastic mantle rock as the openings close, the crust over the source simply continues at its present steady pace towards the convergent trench.
24<9.92 The next expansion cycle will raise the protruding mantle into the underside of the crust again causing it to break while the previously fractured, vertically stressed and laterally tensioned portion aligns to the string of past discharges now trailing beyond the magma source. The newest compromised crust section is then exposed to the high heat of the hotspot fracture's magma. As the cycle repeats the crust is continually moved towards higher temperatures until arriving over the central location. These fractures in the lower crust will slowly open and close as the cycles reoccur.
24<9.93 These fissures are a source of the magma that manifests through the thousands of smaller seafloor mounds and rises. I think these are fed through the untold expansion caused stress fractures that lie beneath the thick layers of ocean floor mud. Many of these lie dormant since the higher thermal event that originally fractured the crust is long over and magma has sealed the crustal fractures. These stress fractures are activated by thermal expansion that extends the crust, reopening the cracks during higher thermal periods. The magma that is delivered beneath the fractures and hotspot/tears will flow out radially under the crust, pressurizing the transitional boundary layer, rising up and supporting the crust during expansion.
24<10.0 The length of the thermal event (the amount of power inducted into the core) determines if the crust's stay over the hot spot is long enough to build a surface anomaly like the Yellowstone complex, an individual volcanic island, or just a short period that produces a small sea mount on the ocean floor. A series of short cooling periods interrupting an overall large thermal period would build a chain of superimposed volcanoes as the crust slowly shifted and the lava discharges intermittently continued. Eventually the Sun's magnetic energy changes and the core begins cooling and as the high heat content of the transitional boundary moves into the crust and ocean, the fissures are slowly closed causing the volcanoes to become dormant. The mantle slowly moves towards the core putting the crust into re-compression against the recent ridge infill. Depending on the timing between thermal cycles and the convergent trenches rate of resistance the crust will shift producing a proportional separation between the islands.
Images above and below courtesy of USGS
24<10.1
Several points to consider;
1. Previous research suggests a location in the center of large ocean basins can promote the development of large, long lasting multi cycle hotspot/mantle-tears.
2. Iceland's Hotspot's long term stationary position on the Atlantic Mid-Oceanic Ridge suggests a hotspot/mantle-tear can precede and promote the initiation of continental break-up process.
3. The Yellowstone hotspot/mantle-tear's periodic progress under the North American continent suggests this Super Volcano was probably born in the Pacific ocean basin and likely formed a volcanic Island chain that has been entirely subducted and/or accreted as the phenomena was overran by North America.
4. Yellowstone will likely proceed on to eventually assist the activity at the New Madrid Seismic Zone, also called the New Madrid Fault Line, a major seismic zone and a prolific source of intraplate earthquakes centered along the Mississippi River.
5. Nascent divergent plate boundaries are likely created in two different scenarios;
a: by catastrophic levels of mantle displacement energies in large continental/oceanic plate assemblies. This scenario is strongly supported by;
such an event occurring at the Middle America Trench (MAT).
https://www.nature.com/articles/s41561-018-0229-y.epdf?referrer_access_token=HnO4WLMkwIDAFKrrT9rJIdRgN0jAjWel9jnR3ZoTv0PCVFi5Qgnv4AZa5anaERJuXVeI0Z9Swq0ea-YUFI2LVQ8XYXvH2evpIz7y0MpjjEksanddQ6LMf6qnf09O8vVzYYHDB5EnBhO9TcYikF3eacXtCXTB4no59PlmIpW7mzk464W0k1PzII2OrWtsMKJLpT438bM9HjQCsqWDqoP98Q%3D%3D&tracking_referrer=www.nationalgeographic.com
Deep embrittlement and complete rupture of the lithosphere during the Mw 8.2 Tehuantepec earthquake
Diego Melgar 1*, Angel Ruiz-Angulo 2, Emmanuel Soliman Garcia 3, Marina Manea 4,5, Vlad. C. Manea 4,5, Xiaohua Xu 6, M. Teresa Ramirez-Herrera 7, Jorge Zavala-Hidalgo 8, Jianghui Geng 9, Nestor Corona 10, Xyoli Pérez-Campos 11, Enrique Cabral-Cano 11 and Leonardo Ramirez-Guzmán 12
24<10.3 "The kinematic slip model of the earth quake challenges current structural models of subduction kinematics; there is normal-faulting (extensional) rupture throughout the entire lithosphere at a point where curvature is high and through portions that should be in flexural compression, and furthermore, rupture spreads well past the 650 °C geotherm (Figs. 1d and 4) into regions where global surveys find that no normal or thrust-faulting intraslab outer-rise-associated seismicity has ever been observed. At the nucleation depth in the bottom half of the oceanic lithosphere, the slab appears to be in tension, contrary to what is predicted from mere flexure"
24<10.4 "Combining these observations, we conclude that, in spite of slab curvature being high (Fig. 3a), the entire lithosphere is under wholesale deviatoric tension, explaining the unusual location of a normal-faulting mechanism in areas expected to be in compression and extending to the base of the lithosphere."
24<10.5 "Thus, combined seismological and geodetic observations of the 2017 Mw 8.2 Tehuantepec, Mexico, earthquake reveal that almost the entire oceanic lithosphere, including the mantle and crust, ruptured in a single large intraslab normal-fault earthquake. It is possible that the 1931 normal-faulting Mw 8.0 Oaxaca earthquake, just east of the Tehuantepec Ridge, similarly broke the entire lithosphere and that both events are part of an ongoing larger-scale breakdown of the slab; however, limited historical seismograms make that determination difficult. Here, we have shown conclusively, with a wide diversity of geophysical observations, that under the right conditions, large earthquakes can nucleate in and rupture through the entire oceanic plate, even for young subduction zones."
24<10.6 b: by hotspot/mantle-tear triple junction initiation and divergent boundary development that sometimes leads to island chain development.
-------------------------------------------------------------------------------------------------------------------------------------------------------
Page 25
Mechanism for
High Speed
Continental Movement
-----------------------------------------------------------------------------------------------------------------------------------------------------------------------------------------------------------------
25<10.7 The model can provide the solution to the perplexing mystery of how the Indian continent was propelled at such a high rate of progress for nearly 75 million years. What the image at the left does not show is how the Indian continent was positioned between two much larger continental plates, the African to its west and the Australian to its east.
25<10.8 These two massively larger plates were in turn positioned against the edge of the even larger Antarctic plate. The boundary where these three plates contacted has a very unique shape, in mechanical engineering it would be described as a compounding mechanism.
25<10.9 The Indian continent's journey occurred over many core thermal cycles/mantle thermal pulses where the tectonic plates were displaced outward by the mantle as the divergent boundaries filled with magma. When the core cooled and the mantle gradually subsided, the most recent infill produced gradually increasing compression in the global plate matrix. As this compression grew the smaller plates were moved at the expense of the larger plate's masses. As the cycles repeated the Indian continent was quickly moved towards Asia.
25<10.11 http://www.researchgate.net/publication/10736864_Bonatti_E._et_al._Mantle_thermal_pulses_below_the_Mid-Atlantic_Ridge_and_temporal_variations_in_the_formation_of_oceanic_lithosphere._Nature_423_499-505
Mantle thermal pulses below the Mid-Atlantic Ridge and temporal variations in the formation of oceanic lithosphere
25<10.12 "A 20-Myr record of creation of oceanic lithosphere at a segment of the central Mid-Atlantic-Ridge is exposed along an uplifted sliver of lithosphere. The degree of melting of the mantle that is upwelling below the ridge, estimated from the chemistry of the exposed mantle rocks, as well as crustal thickness inferred from gravity measurements, show oscillations of ,3–4 Myr superimposed on a longer-term steady increase with time. The time lag between oscillations of mantle melting and crustal thickness indicates that the solid mantle is upwelling at an average rate of ,25mmyr, but this appears to vary through time."
25<10.13 So, we now need direct evidence that these cycles appear in the observational research of the Indian Continent's progress.
https://www.science.org/doi/10.1126/sciadv.aaz8681
The convergence history of India-Eurasia records multiple subduction dynamics processes
ADINA E. PUSOK HTTPS://ORCID.ORG/0000-0002-4439-8124 AND DAVE R. STEGMAN
25<10.14 RESULTS
25<10.15 It's difficult to ignore this model's repeated abilities to predict this planet's operational parameters.
25<10.21 Is there any evidence that the Indian continent could have been compressed between two larger continents and squeezed out from between them? Leaving the two opposing plate sections with deformities that exhibit the telltale signs that their plate edges were subjected to great pressures?
25<10.3 The adjacent plate sections exhibit such deformities where India appears to have traveled between them.
Image above was modified by this author and was furnished through and in no way endorsed by http://www.geomapapp.org using Global Multi-Resolution Topography (GMRT) Synthesis,
Ryan, W. B. F., S.M. Carbotte, J. Coplan, S. O'Hara, A. Melkonian, R. Arko, R.A. Weissel, V. Ferrini, A. Goodwillie, F. Nitsche, J. Bonczkowski, and R. Zemsky (2009), Global Multi-Resolution Topography (GMRT) synthesis data set, Geochem. Geophys. Geosyst., 10, Q03014, doi:10.1029/2008GC002332.Data doi: 10.1594/IEDA.0001000, through http://creativecommons.org/licenses/by-nc-sa/3.0/us/
25<10.4 This seafloor age map below gives a more definitive idea of where the likely path was, undoubtedly between the pressure ridges. During the intervening years seafloor has been added during expansion portion of the cycle as the Indian continent was slowly forced northward by the unimaginable energy furnished by the mantle's return towards the core.
Credit for images above: Mr. Elliot Lim, CIRES & NOAA/NGDC, and citation to Müller, R.D., M. Sdrolias, C. Gaina, and W.R. Roest 2008. Age, spreading rates and spreading symmetry of the world's ocean crust, Geochem. Geophys. Geosyst., 9, Q04006, doi:10.1029/2007GC001743.
-----------------------------------------------------------------------------------------------------------------
Page 26
Mechanism for
Mountain Formation
26<10.5 This mechanism is the long sought builder of mountain ranges around the Earth. The mystery of how and why colliding land masses don't halt but continue to move into the only unobstructed direction, skyward, beyond any theorized energy source's ability to not only produce but deliver the required traction to compress, fold, override, and raise 8.8 meters (5.5 miles) into the atmosphere a chain of mountains has gone until now unanswered in current plate dynamics theories. But because all of the Earth’s divergent plate boundaries find magma infill simultaneously, the tectonic plates are all preloaded together creating a continuous unmovable foundation for the tectonic plates to convert their mass weight from vertical gravitational energy to horizontal kinetic energy to move the plates in the path of least resistance whether it be an oceanic plate subducting, a continental plate overriding another, or the mass collision of rock driven vertically into a mountain range.
Image below courtesy NASA Ames Research Center, World Wind. Himalayas as pictured by NASA Landsat 7 Satellite.

Gravitational potential energy of the Tibetan Plateau and the forces driving the Indian plate
Attreyee Ghosh, William E. Holt
Department of Geosciences, State University of New York, Stony Brook, New York 11790, USA
Lucy M. Flesch*
Department of Terrestrial Magnetism, Carnegie Institution of Washington, Washington, DC 20015, USA
A. John Haines†
Bullard Laboratories, University of Cambridge, Cambridge CB3 0EZ, UK
ABSTRACT
"We present a study of the vertically integrated deviatoric stress field for the Indian plate and the Tibetan Plateau associated with gravitational potential energy (GPE) differences. Although the driving forces for the Indian plate have been attributed solely to the mid-oceanic ridges that surround the entire southern boundary of the plate, previous estimates of vertically integrated stress magnitudes of 6–7 1012 N/m in Tibet far exceed those of 3 1012 N/m associated with GPE at mid-oceanic ridges, calling for an additional force to satisfy the stress magnitudes in Tibet. We use the Crust 2.0 data set to infer gravitational potential energy differences in the lithosphere. We then apply the thin sheet approach in order to obtain a global solution of vertically integrated deviatoric stresses associated only with GPE differences. Our results show large N-S extensional deviatoric stresses in Tibet that the ridge-push force fails to cancel."
26<10.64 . . . . "However, the ridge push, or vertically integrated deviatoric stress magnitude, which is 3 1012 N/m (Richardson, 1992; Harper, 1975; Lister; 1975; Parsons and Richter, 1980), is not sufficient to satisfy inferred stress magnitudes of 6–7 1012 N/m that result from GPE differences between the Tibetan Plateau and the surrounding lowlands (Molnar and Lyon-Caen, 1988). An additional force is required to explain the disparity between the excess GPE of Tibet relative to that of the mid-oceanic ridges" . . . .
26<10.65 . . . ."Lithospheric density variations associated with the support of the high topography of the Tibetan Plateau give rise to lithospheric body forces and hence stresses. Although the sources of stress that drive plate motions have been ascribed to many parameters (Forsyth and Uyeda, 1975), from the point of view of stress continuity and force balance, the stresses that drive lithospheric motion arise from two sources: (1) gravity acting on density variations within the lithospheric shell on Earth, and (2) gravity acting on density variations deeper than the lithospheric shell. The latter gives rise to tractions (radial and tangential) that act on the base of the lithosphere, affecting the stress field of the lithosphere and producing dynamic topography.The former involves density variations associated with support of nondynamic components of topography".
Conclusions;
. . "It is clear that something is missing as a driving force that does not have its source within the lithospheric shell."
26<10.8 The African and Australian continental plates are moving to the northeast. The Indian plate lies at the pinch point of these two powerful traction engines. Their combined gravitationally driven lateral forces being concentrated into the comparative narrow area of convergence of the two continental plates propels India like a prow of trireme into the side of Asia.
The African and Australian plate's Northern convergent zones have outside of this convergence with India, mostly subducting or fault slip oceanic/continental shelf that has appreciably less resistance allowing a geologically continuous mechanical advancement.
Image above was furnished through and in no way endorsed by http://www.geomapapp.org using Global Multi-Resolution Topography (GMRT) Synthesis,
Ryan, W. B. F., S.M. Carbotte, J. Coplan, S. O'Hara, A. Melkonian, R. Arko, R.A. Weissel, V. Ferrini, A. Goodwillie, F. Nitsche, J. Bonczkowski, and R. Zemsky (2009), Global Multi-Resolution Topography (GMRT) synthesis data set, Geochem. Geophys. Geosyst., 10, Q03014, doi:10.1029/2008GC002332.Data doi: 10.1594/IEDA.0001000, through http://creativecommons.org/licenses/by-nc-sa/3.0/us/
26<10.9 I was studying some controversy in regards to mountain formation. There is a great paper that outlines the theory that proposes a more recent and defined era of rapid mountain building on a global scale.
ANNALS OF GEOPHYSICS, SUPPLEMENT TO VOL. 49, N. 1, 2006
Mountain uplift and the Neotectonic Period
CLIFF D. OLLIER
9.2. EXAMPLES
9.2.1. Tibet, Himalayas, Kunlun Mountains
(As an example, consider the timing of uplift in Tibet and its bordering mountains. Gansser (1991) wrote: «... we must realize that the morphogenic phase is not only restricted to the Himalayas but involves the whole Tibetan block. This surprising fact shows that an area of 2500000 km2 has been uplifted 3000-4000 m during Pleistocene time and that this uplift is still going on.» In places the uplift rate is 4.5 mm/yr (five times the maximum in the European Alps). According to Wu et al. (2001) from the Pliocene to the Early Quaternary (5-1.1 Million years) the Kunlun Pass area of the Tibetan Plateau was no more than 1500 m high and was warm and humid. They write: «The extreme geomorphic changes in the Kunlun Pass area reflect an abrupt uplift of the Tibet Plateau during the Early and Middle Pleistocene. The Kunlun-Yellow River tectonic movement occurred 1.1-0.6 Million years.» Zheng et al. (2000) concluded from sediments at the foot of the Kunlun Mountains that uplift began around 4.5 Million years.)
9.4. CONCLUSIONS
(Mountains are created by the vertical uplift of former plains, independent of any folding of the rocks underneath. The age of mountains should therefore refer to the age of vertical uplift after planation, not to the last period of folding (if the underlying bedrock happens to be folded). Most uplift occurred in the Plio-Pleistocene, or the very Late Miocene. The Neotectonic Period is demonstrated by the large amount of work listed in table 9.I. Plate tectonics, the ruling theory of the past forty years, has no adequate explanation for the widespread planation in mountain regions, or the remarkably young uplift. Indeed it is based on an association of folding and uplift that is demonstrably untrue. Plate tectonics has no plausible explanation for mountains on passive margins or continental interiors. From now on it is incumbent on those who propose models of mountain formation to do two things: Incorporate planation surfaces into the story (or prove there was no former planation). – Either disprove the Neotectonic Period hypothesis, or show how their proposed mechanisms fit into the time scale of just a few million years). . . . .(Uplift occurred over a relatively short and distinct time. Some unknown process created mountains after a period with little or no significant uplift. This is a deviation from uniformitarianism. The mountain building period is relatively short, and not on the same time scale as granite intrusion (which takes tens of millions of years), or plate tectonics which is supposedly continuous over hundreds of millions of years. The same rapid uplift occurs in areas where hypotheses such as mantle plumes are not appropriate. We do not yet know what causes this short, sharp period of uplift, but we can exclude naive mountain-building hypotheses that are on the wrong time scale.) . . .(One of the biggest obstacles to our hypothesis of widespread Plio-Pleistocene mountain building is that the period of time available for the preceding planation is too short. Only further investigation can clarify this point.)
26<11.0 The variability of the mantle thermal pulses can answer this challenge. I believe long and short durational periods of high magnetic field induction have driven various geologic events, the breakup of Pangaea and the subsequent 600 thousand year cataclysmic volcanic event for one. I want to check another source.
http://pubs.usgs.gov/gip/dynamic/himalaya.html
26<11.1 "The collision of India into Asia 50 million years ago caused the Indian and Eurasian Plates to crumple up along the collision zone. After the collision, the slow continuous convergence of these two plates over millions of years pushed up the Himalayas and the Tibetan Plateau to their present heights. Most of this growth occurred during the past 10 million years. The Himalayas, towering as high as 8,854 m above sea level, form the highest continental mountains in the world. Moreover, the neighboring Tibetan Plateau, at an average elevation of about 4,600 m, is higher than all the peaks in the Alps except for Mont Blanc and Monte Rosa, and is well above the summits of most mountains in the United States."
26<11.13 This sudden mountain building energy and era is seen in the South American Andes as well;
http://www.rochester...n-in-the-andes/
26<11.14 “This study provides increasing evidence that the plateau formed through periodic rapid pulses, not through a continuous, gradual uplift of the surface, as was traditionally thought,” said Garzione. “In geologic terms, rapid means rising one kilometer or more over several millions of years, which is very impressive.”
26<11.15 “What we are learning is that the Altiplano plateau formed by pulses of rapid surface uplift over several million years, separated by long periods (several tens of million years) of stable elevations,” said Garzione.
26<11.2
http://www.rochester.edu/news/show.php?id=3167
26<11.21 “Tectonic Theory May Need Revision in Light of New Study in Science”
“Mountains may experience a "growth spurt" that can double their heights in as little as two to four million years—several times faster than the prevailing tectonic theory suggests.”
26<11.22 “By studying sedimentary basins in the high Andes Mountains, the team could determine when and at what altitude these ancient sediments were deposited. That record of altitude changes shows that the Andes Mountains rose slowly for tens of millions of years, but then suddenly lifted much faster between 10 and 6 million years ago.”
26<11.3 Stunning isn't it. The two youngest and largest ranges by way of their own metrics occurred simultaneously. Here we have not one but two examples of massive energy dispositions in a geologic blink of an eye in opposite hemispheres at the same time. And the standard model would prescribe two different causes no doubt despite the rather devastating evidence regarding the conclusion earlier;
26<11.31 http://pubs.usgs.gov...c/himalaya.html
"It is clear that something is missing as a driving force that does not have its source within the lithospheric shell."
26<11.32 The time period is short, again, geologically in a blink of an eye. If their hypothesis is correct the mountain building periods have occurred repeatedly over the Earth's history. These mountains are surely not a onetime phenomena. But the periods in between have to be long enough to allow the former to erode substantially.
26<11.4 The mechanical advancement of Indian plate in this model has the required energy to raise and hold the Tibetan Plateau. The model has the Sun in an extended magnetic field energy period. As the thermal expansion cycle begins, the mantle's slow release of hydraulic magma presses out the Earth’s crust and all divergent plate boundaries open and receive magma infill. This period of high magnetic energy will slowly raise the core temperature, causing the slow and gradual displacement of the crust and mantle. The extensional processes will produce the planation through the resistance to allowable movement by the heavier sections of crust. This frictional drag stretches the lithosphere and extends upper crust, lowering the surface layers into the thinning substrate. The expansion period is long (2-3 million + years) and mostly in one direction (expansion) with a few lapses in solar magnetic energy that would allow cooling in the crust/mantle boundary and short episodes of increased movement and earthquakes in the subduction zones of the crust.
26<11.41 This unusually long period of expansion has now ended, but it has raised the billion trillion ton core's temperature a substantial amount and now as that heat dissipates the cycle then switches to the thermal contraction phase when the crust/mantle boundary begins to cool. All the Earth's tectonic plates are then put into compression against the new magma infill and convert their vertical potential energy through gravitational force into the kinetic path of least resistance. As these cycles repeat they will either add gradually increasing or decreasing Gravitational Potential Energy content into the crust depending on the overall net plus gain or loss of ridge infill.
Department of Geological Sciences, Brown University, Providence, Rhode Island
26<11.52 "As most of the oceanic lithosphere is in a state of net compression, the question arises as to why intraplate deformation has developed in these regions and not in others."
26<11.53 The models ability to raise the global tectonic plate matrix while shoring the retreating divergent plate boundaries with new magma provides a means where the initial thermal expansion energy can be stored in the now increasingly compressed and increasingly raised mass of the crust as (short term) gravitational potential energy then slowly released as kinetic energy as the plates melt into the asthenosphere. Periods of excessive gravitational potential energy, the periods that exceed the trenches rates of resistance will produce (long term) storage of the kinetic energy as mass in mountain complexes.
-----------------------------------------------------------------------------------------------------------------
Page 27
Model for the creation, storage and distribution of:
Compression and Gravitational Potential Energy
Within the Crust
------------------------------------------------------------------------------------------------------------------------------------------------------------------------------------------------------------------
27<11.61 In the heat cycle there are several variables to consider, one is the amount of outer core thermal expansion energy that can be delivered to the mantle in the form of mechanical expansion (displacement). How much can the mantle be displaced in one cycle? Another is how variable is the timing between expansion periods and the degree to which the mantle subsides between them, this determines how much gravitational potential energy can be realized in the crust. How much will the crust store and how much of that energy will it release between cycles?
27<11.62
http://www.researchgate.net/publication/10736864_Bonatti_E._et_al._Mantle_thermal_pulses_below_the_Mid-Atlantic_Ridge_and_temporal_variations_in_the_formation_of_oceanic_lithosphere._Nature_423_499-505
Mantle thermal pulses below the Mid-Atlantic Ridge and temporal variations in the formation of oceanic lithosphere
27<11.63 "A 20-Myr record of creation of oceanic lithosphere at a segment of the central Mid-Atlantic-Ridge is exposed along an uplifted sliver of lithosphere. The degree of melting of the mantle that is upwelling below the ridge, estimated from the chemistry of the exposed mantle rocks, as well as crustal thickness inferred from gravity measurements, show oscillations of ,3–4 Myr superimposed on a longer-term steady increase with time. The time lag between oscillations of mantle melting and crustal
thickness indicates that the solid mantle is upwelling at an average rate of ,25mmyr, but this appears to vary through time."
27<11.7 These factors determine whether the crust will build up a net plus of compressive energy. The subduction of the crust may take longer than it took the ridge to produce it. Since subduction requires the plate to be driven into the substrate continually, the core temperature decrease that initiated the increase in subduction is now the moderator of its decline. The lower the mantle temperature the slower the crust will move into the convergent boundary. The compression will build in proportion to the temperature decrease and mantle contraction while the duration of compression will be extended by the resistance of the cooler convergent boundary.
27<11.71 The compressive energy can be stored and then delivered in a geologically fast period of time because the subduction rate lags behind the contraction of the mantle. During increasing solar magnetic field energy, the crust is continually pressed out to allow more infill of magma that in turn creates more future potential compression during the later contraction. This is the mechanism that the before mentioned paper alludes to. “Either disprove the Neotectonic Period hypothesis, or show how their proposed mechanisms fit into the time scale of just a few million years”. The degree and time span of the solar magnetic energy is directly proportionate to the amount of potential gravitational driven kinetic energy stored in the crust.
27<11.8 The model's mechanism can operate, due to low solar magnetic energy, at a rate of slow and very low kinetic energy plate movement. The small movement in the crust from the cooler mantle cycles allows only small infills of magma which produce very small horizontal movement. The lowest thermal cycles may even be lower than the subduction trench's lowest rate of resistance.
27<11.81 The compressive energy of the global plate matrix during these periods cannot produce any mountain systems. Any compressive plate load would easily bleed into the convergent plate boundaries before any continent to continent collisions would even dent each other. Compare this example to the mountain building period. The tectonic plates are expanded and there boundaries infilled with magma, building a future compression surplus, increasing the potential kinetic energy beyond the subduction trenches rates of resistance. During the contraction phase the trenches begin to resist any further increase in plate subduction.
27<11.82 As the compressive energy slowly builds in the crust from the cooling core and receding mantle the continental inter-plate zones like the Himalayas receive a continual buildup of compression. At some point the subduction trenches' resistance to the compression causes a sudden direction of force change in the subducting plate, it moves in the direction of least resistance, upwards raising the overriding land mass in a geologically fast period of time. The rise is probably faster at the beginning from the initial release of compressive energy to the vertical direction. The rise is only the precursor to what will be a geologically rapid (less than 2 million years) direction of force change in the hyper-compressed substrate of the plate's rock strata resulting in vertical rupture and folding of the plate. This folding is rapid due to the large amount of potential kinetic energy still unloading from the plate matrix. This variable in plate dynamics will undoubtedly require a reexamination of geological timelines based on ridge infill / sea floor spreading.
27<12.0 There have been questions raised that the trenches could not receive the volume of crust produced. But the volume of the subduction is determined by the GPE stored in the raised crust, the trenches rate of resistance and the length of the contraction period, all of which happens long after the mantle has stopped expanding (displacing) and time will be the mediator to the processes that determine the ocean plate’s creation and its eventual destruction.
27<12.1 I believe the crust and mantle have been in a expansion mode almost the entire interglacial. And when the surface of the mantle starts to contract and then the ocean begins to cool and the crust changes from this current period where there is high levels of solar magnetic energy and divergent boundary movement to when there are lower levels of magnetic energy, and the crust is acquiring even more compression with much less or even possibly not any divergent movement, and as the trenches begin to receive what will be a long period of increased compressional energy in the crust, the next glacial will be under way.
27<12.2 The glacial periods last approximately 100,000 years and are likely to see increased mantle driven contraction. The million + years worth of gravitational potential energy stored in the crust will overlap the glacial and slowly produce or simply increase compression into the trenches throughout these transient thermal periods.
27<12.21 At these time scales there is just the disposition of heat or the lack there of. During these periods any solar magnetic levels that resulted in thermal expansion in the core would manifest as small temperature gains in the crust/mantle boundary as the mantle's surface is forced to decompress by mechanical expansion, resulting in a temperature gain as ocean water thermally warms as the boundary displacement is activated from below, similar to what is currently being observed taking place at this time at the West Antarctic Rift System that has slowly but steadily reactivated since at least the last decade of the last century.
27<12.22
https://en.wikipedia.org/wiki/West_Antarctic_Rift_System
https://phys.org/news/2014-06-major-west-antarctic-glacier-geothermal.html
1. Researchers find major West Antarctic glacier melting from geothermal sources
"According to his findings, the minimum average geothermal heat flow beneath Thwaites Glacier is about 100 milliwatts per square meter, with hotspots over 200 milliwatts per square meter. For comparison, the average heat flow of the Earth's continents is less than 65 milliwatts per square meter."
https://www.nsf.gov/news/news_summ.jsp?cntn_id=295861&org=NSF&from=news
2. Previously unsuspected volcanic activity confirmed under West Antarctic Ice Sheet at Pine Island Glacier
""Our finding of a substantial heat source beneath a major WAIS glacier highlights the need to understand subglacial volcanism, its interaction with the marine margins and its potential role in the future stability of the WAIS,” they write in the Nature Communications article.""
"They also note that volcanic activity could be increasing the rate of collapse of the Thwaites Glacier, which is adjacent to the Pine Island Glacier.''
"A complete collapse of the Thwaites Glacier could significantly affect global sea levels, according to scientists. The Thwaites already drains an area roughly the size of the state of Florida, accounting for about 4 percent of global sea level rise -- an amount that has doubled since the mid-1990s."
https://sp.lyellcollection.org/content/specpubgsl/early/2017/05/26/SP461.7.full.pdf
3. A new volcanic province: an inventory of subglacial volcanoes in West Antarctica
"West Antarctica hosts one of the most extensive regions of stretched continental crust on the Earth, comparable in dimensions and setting to the East African Rift System and the western USA’s Basin and Range Province"
https://pubs.geoscienceworld.org/gsa/geosphere/article/14/6/2407/565649/Geodynamic-models-of-the-West-Antarctic-Rift
4. Geodynamic models of the West Antarctic Rift System: Implications for the mantle thermal state
https://agupubs.onlinelibrary.wiley.com/doi/pdf/10.1002/2017GL075609
5. Heat Flux Distribution of Antarctica Unveiled
https://www.theguardian.com/world/2017/aug/12/scientists-discover-91-volcanos-antarctica
6. Scientists discover 91 volcanoes below Antarctic ice sheet
"After the team had collated the results, it reported a staggering 91 previously unknown volcanoes, adding to the 47 others that had been discovered over the previous century of exploring the region."
"The project, by Edinburgh University researchers, has revealed almost 100 volcanoes – with the highest as tall as the Eiger, which stands at almost 4,000 metres in Switzerland."
"Geologists say this huge region is likely to dwarf that of east Africa’s volcanic ridge, currently rated the densest concentration of volcanoes in the world."
27<12.23 It is quite significant that this sudden increased activation of the planet's largest active volcanic province containing the planet's largest active rifting zone has occurred simultaneously with the climate change phenomenon.
27<12.3 "Primarily" is referring to the variability in this phenomena, the thermal component is a constant variable. Even though the mantle is slowly moving out during an overall period of an outward oscillation phase that lasts for several million years, there would be short and longer pulses repeatedly for hundreds of thousands of years, it would have continuous variability in core temperature influencing its rate and temperature. It may cool slightly and slow periodically for periods of varying lengths.
27<12.31 The Little Ice Age for example, would be the most likely effect of a cooling of this nature. Another possible response to mantle cooling is the Younger Dryas stadial, sometimes called the Big Freeze; it was a geologically brief (1,300 ± 70 years) period of cold climatic conditions that occurred between approximately 12,800 and 11,500 years before present.
27<12.32 Data from Greenland ice core GISP2 indicate that Greenland was approximately 15 °C (27.0 °F) 59° colder during the Younger Dryas than today - (Alley, Richard B. et al. (1993). "Abrupt accumulation increase at the Younger Dryas termination in the GISP2 ice core". Nature 362) (6420): 527–529.
27<12.33 Nothing of the size, extent, or rapidity of this period of abrupt climate change has been experienced since. - (Alley, Richard B. (2000). "The Younger Dryas cold interval as viewed from central Greenland". Quaternary Science Reviews 19 (1): 213–226.)
27<12.4 The mountain building period mentioned above began just before the current 2.58 million year long ice age period. It seems the mountain building period was concurrent with cooler climate periods. Any time before an ice age is warmer but I would expect a period that has mountain building to have a long period of higher core temperatures before it. While periods of higher compressive plate energies would be expected to extend into the midst of a glacial period. This increase of compression may account for some Island Arc volcanism near the trenches during these colder periods as the plates continue to unload large inventories of gravitational potential energy. For example; Lake Toba supervolcano erupted 75,000 years before present, causing a volcanic winter that pushed some species including humans to the brink of extinction.
-----------------------------------------------------------------------------------------------------------------
Page 28
Model for Globally Simultaneous Boundary Deformation
with subsequent and significant
Carbon Isotope Excursions and
Mass Extinctions (Evolutionary Mechanism)
--------------------------------------------------------------------------------------------------------------------------------------------------------------------------------------------------------------------------------------------------------------------------------
28<16.1 This model proposes that all tectonic boundaries are simultaneously loaded with compression derived from the gravitational potential energy produced by the mantle’s periodic subsidence. And that the boundaries current physical configurations belie their disposition of, and response to, the highest levels of GPE present during those periods that are geographically and temporally simultaneous.
28<16.2 The Himalayan and Andean ranges have been shown to be geologically synchronous in origin and that their source and timing are both dependent on the same mechanism this model proposes. This source’s simple surface mechanics that explain so well the origin of these ranges must also impose itself on all other boundaries to proportionate degrees.
28<16.21 These boundaries must include the divergent Mid Ocean Ridges as well. Taking into account their own and very unique geologic circumstances they should tell the story of their physical arrangement and place within the reference frame of the Plio-Pleistocene Mountain building era as proposed by Dr. Ollier and others.
28<16.22 The oceanic plates in question, the Pacific and Atlantic, can be analyzed using this model to reveal their responses to the energies disposed onto them, revealing the exact nature of the mountain building event in a global scale reference frame.
28<16.23 The image below is a typical Pacific Mid Ocean Ridge cross section taken west of Ecuador. Please note the base width is approximately 25-26 km or around 16 miles and the height is 500 m. or 1640 ft.
Images and cross sections above and those below were furnished through and in no way endorsed by http://www.geomapapp.org using Global Multi-Resolution Topography (GMRT) Synthesis,
Ryan, W. B. F., S.M. Carbotte, J. Coplan, S. O'Hara, A. Melkonian, R. Arko, R.A. Weissel, V. Ferrini, A. Goodwillie, F. Nitsche, J. Bonczkowski, and R. Zemsky (2009), Global Multi-Resolution Topography (GMRT) synthesis data set, Geochem. Geophys. Geosyst., 10, Q03014, doi:10.1029/2008GC002332.
Data doi: 10.1594/IEDA.0001000, through http://creativecommons.org/licenses/by-nc-sa/3.0/us/
29<16.3 Compare the Pacific MOR above to this one below of a typical Atlantic cross section.
29<16.4 The 26 km Pacific MOR is dwarfed by its Atlantic counterpart, the Atlantic’s spreading center at this location is typical of the entire range in that it resembles a proto basin, here it is over 8 km wide. Below is a 140 km wide Atlantic cross section image.
29<16.6 And finally at 550 km image, notice also it rises 2400 m., or 1.5 miles, as compared to the Pacific MOR at its much lower 500 m.
29<16.7 It is remarkable how physically different these two boundaries appear in these cross sections. The spreading center of the Pacific MOR is diminutive against the Atlantic’s.
Now let’s look at an overhead view of these two spreading centers, first the Pacific.
29<16.91 The standard model explains that this is due to the mantle under the Atlantic ridge having higher temperatures, and by that lower densities, leading to the formation of a bulge at the mantle surface area directly below the ridge, and this has led to the crust above to be formed at a higher topography than the adjacent and presumed cooler mantle areas to either side.
29<16.92 This is typical of the standard model, each phenomena has a custom rendered thermal dependent answer based on an upward convective force of one type or another.
29<17.0 This model can reconstruct the circumstances leading up to this phenomena and show it is simply the result of its current position within its own developmental timeline.
29<17.1 This model proposes the elevation of the Mid Atlantic Ridge, or MAR, is the result of the Pleo-Pleistocene mountain building era and can be shown to fit perfectly to that timeline and the surface mechanics that this model confidently exhibits for those and other geologic phenomena.
29<17.11 We can start this examination by looking at the paper by Dr. Ollier again;
ANNALS OF GEOPHYSICS, SUPPLEMENT TO VOL. 49, N. 1, 2006
Mountain uplift and the Neotectonic Period
CLIFF D. OLLIER
School of Earth and Geographical Sciences, University of Western Australia, Perth, Australia
9.2. EXAMPLES
9.2.1. Tibet, Himalayas, Kunlun Mountains
Uplift began at 4.5 MYA.
29<17.13 Below are some additional cross sections along the Atlantic ridge like those shown above. And taking into consideration that their data has also been laterally compacted and the actual basin that is developing between the ridges is much wider than shown. For example in the image below the ridge on the right is over 2 km high while the spreading center basin that lies between the ridges is around 9 km wide. The basin therefore would need to be shown as being more than 4 times wider to be in proper scale to the ridge elevation. This model proposes that before the Pleo-Pleistocene the Atlantic boundary exhibited much lower elevations, ones closer to those of the Pacific MOR.
29<17.2 The distance between the highest points of the opposing ridges average around 50km +/- 10km or so. Taking the given rate of ridge movement from;
29<17.21
http://pubs.usgs.gov...erstanding.html
29<17.22 "The rate of spreading along the Mid-Atlantic Ridge averages about 2.5 centimeters per year (cm/yr), or 25 km in a million years."
29<17.23 It would then seem possible that if the geologic clock were to be ran backwards, and the last 2 million years of divergent boundary infill was removed, we would be observing a combination of the two ridges into a summit resembling one similar to that of the Pacific, with a spreading center that was substantially more in alignment with its summit than the current very low basin that now occupies the area where the actual boundary is currently separating.
29<17.3 This model proposes that the same GPE derived compression that raised so much crustal mass into ranges around the world during the Pleo-Pleistocene era did the same to this boundary. And if that compression could have been diverted into a compression relieving mechanism such as a convergent trench then the Atlantic ridge would more resemble the Pacific’s very small summit profile while the overall structure would be reduced substantially to one resembling the Pacific’s mere 500 m. elevation.
29<17.4 The circumstances involved in the development of the Atlantic ridge can be determined by the way these two boundaries responded to that geologic event that formed the Himalayas, the Andes and many other ranges several million years ago. The mountain building era requires a prior period of almost continual outward mantle displacement to put in place an unusually large inventory of divergent boundary material.
29<17.41 I am referring to a specific period of time several million years ago when mountain ranges around the world suddenly rose up. These mountains are on continental to continental boundaries (Himalayas), oceanic to continental boundaries (Andes), and as I'm claiming here, oceanic to oceanic boundaries (Mid Atlantic Ridge). I can show the Atlantic's exceptional size (and yes it is exceptional compared to all other MORs) is the result of the same forces as those other mountain ranges mentioned, and others not mentioned but date to the same time period. So this idea is about globally dispersed boundary deformation that took place around 2-4 million years ago. The reasons why some boundaries produced mountains and others didn't will hopefully be explained here.
29<17.42 “According to Wu et al. (2001) from the Pliocene to the Early Quaternary (5-1.1 Million years) the Kunlun Pass area of the Tibetan Plateau was no more than 1500 m high and was warm and humid.”
29<17.5 The time frame I’m discussing here begins at that time of the Pliocene and extends into the Pleistocene where most of this mountain uplift was largely completed. This was a massive expenditure of energy in an incredibly short period of time. And it occurred in both hemispheres and multiple continents all at the same time. Does anyone find this more than a little challenging for the standard model to explain?
29<17.51 OK, back to square one.
Let’s imagine that the new divergent boundary infill that is currently being placed in all the world’s MORs is due to the Earth’s crust is being simultaneously moved outward by the mantle oscillations as described by;
29<17.53 "A 20-Myr record of creation of oceanic lithosphere at a segment of the central Mid-Atlantic-Ridge is exposed along an uplifted sliver of lithosphere.The degree of melting of the mantle that is upwelling below the ridge, estimated from the chemistry of the exposed mantle rocks, as well as crustal thickness inferred from gravity measurements, show oscillations of ,3–4 Myr superimposed on a longer-term steady increase with time.The time lag between oscillations of mantle melting and crustal thickness indicates that the solid mantle is upwelling at an average rate of ,25mmyr, but this appears to vary through time."
29<17.54
The four important clues that stand out to me and can be correlated to this model are;
1.-A 20-Myr record of creation of oceanic lithosphere
2.-oscillations of ,3–4 Myr
3.-longer-term steady increase with time
4.-the solid mantle is upwelling at an average rate of ,25mmyr, but this appears to vary through time."
29<17.6 Now let’s look at a simple dynamic model of the earth’s crust that resembles what the convergent and divergent boundaries looked like prior to that period described by Dr. Ollier below;
ANNALS OF GEOPHYSICS, SUPPLEMENT TO VOL. 49, N. 1, 2006
Mountain uplift and the Neotectonic Period
CLIFF D. OLLIER
School of Earth and Geographical Sciences, University of Western Australia, Perth, Australia
9.2. EXAMPLES
9.2.1. Tibet, Himalayas, Kunlun Mountains
29<17.61 (As an example, consider the timing of uplift in Tibet and its bordering mountains. Gansser (1991) wrote: «... we must realize that the morphogenic phase is not only restricted to the Himalayas but involves the whole Tibetan block. This surprising fact shows that an area of 2500000 km2 has been uplifted 3000-4000 m during Pleistocene time and that this uplift is still going on.» In places the uplift rate is 4.5 mm/yr (five times the maximum in the European Alps).
According to Wu et al. (2001) from the Pliocene to the Early Quaternary (5-1.1 Million years) the Kunlun Pass area of the Tibetan Plateau was no more than 1500 m high and was warm and humid. They write: «The extreme geomorphic changes in the Kunlun Pass area reflect an abrupt uplift of the Tibet Plateau during the Early and Middle Pleistocene. The Kunlun-Yellow River tectonic movement occurred 1.1-0.6 Million years.» Zheng et al. (2000) concluded from sediments at the foot of the Kunlun Mountains that uplift began around 4.5 Million years.)
29<17.7 And consider these studies of the Andes also;
http://www.rochester...n-in-the-andes/
29<17.71 “This study provides increasing evidence that the plateau formed through periodic rapid pulses, not through a continuous, gradual uplift of the surface, as was traditionally thought,” said Garzione. “In geologic terms, rapid means rising one kilometer or more over several millions of years, which is very impressive.”
29<17.72 “What we are learning is that the Altiplano plateau formed by pulses of rapid surface uplift over several million years, separated by long periods (several tens of million years) of stable elevations,” said Garzione.
29<17.73
http://www.rochester...how.php?id=3167
29<17.74 “Tectonic Theory May Need Revision in Light of New Study in Science”
“Mountains may experience a "growth spurt" that can double their heights in as little as two to four million years—several times faster than the prevailing tectonic theory suggests.”
29<17.75 “By studying sedimentary basins in the high Andes Mountains, the team could determine when and at what altitude these ancient sediments were deposited. That record of altitude changes shows that the Andes Mountains rose slowly for tens of millions of years, but then suddenly lifted much faster between 10 and 6 million years ago.”
29<17.8 This mountain building in the Himalayas, Andes and other locations started with raising large areas of much lower land simultaneously around the world in very short periods of time.
29<17.81 If we could imagine that the crust could supply its own energy to do this by utilizing the GPE it attains when the mantle changes from when 4.-“the solid mantle is upwelling at an average rate of ,25mmyr” to when it moves down once again.
29<17.82 The GPE in the crust would then increase because the divergent boundary infill in all boundaries from the last “upwelling” phase of the cycle would need to push the plates towards a convergent trench to equalize the growing compressive energy that the most recent infill is now being subjected to and is passing on to all the planet’s plates.
29<17.9 We should then be able to look at the boundaries around the world, both convergent and divergent, and see evidence of their interaction with this last episode of higher GPE that occurred during the Plio-Pleistocene mountain building era.
29<17.91 An important point to remember is; if the crust that is adjacent to the boundary (convergent or divergent) is unable to move towards a lower energy state that is at a level of resistance low enough to process the mantle’s rate of subsidence, then the boundary, whether it is convergent or divergent, will be subjected to higher compressive energies.
29>18.1 This image above represents a divergent boundary similar to the Pacific’s. If we were to imagine the mantle under it had begun moving downward the two continents would begin to move towards each other, and because the world is a sphere they would move towards all the other continents as well.
29>18.2 The opposing oceanic plates that are portrayed in the image would begin to compress their divergent boundary. But the resulting compression could only reach to the level that the subducting crust encounters at the opposing convergent trenches. The trenches behave as compression relieving mechanisms and limit the amount of compression the divergent boundary can be subjected to.
29<18.21 This illustration above represents a combined continental/oceanic spreading center arrangement similar to that of the Atlantic’s. The oceanic crusts are attached to their parental continents as they have been since they first divided. As the two continents move towards each other the convergent boundary is loaded with compression, the oceanic plates respond to this in the only way they can, by pushing back.
29>18.22 In this scenario it would be reasonable to expect the Atlantic boundary, in not having the compression relieving mechanisms of convergent boundary trenches, would experience higher levels of compression then the Pacific would.
29>18.23 When there is (1).-“A 20-Myr record of creation of oceanic lithosphere” with (2).-“oscillations of ,3–4 Myr”, during which they have a (3).-“longer-term steady increase with time” during which (4).-“the solid mantle is upwelling”; the following subsidence period would have a larger portion of GPE in the crust.
29>18.24 A “steady increase with time” over a period of 20 million years would leave a gradually building inventory of surplus divergent boundary material to be dealt with. When the “20 Myr increase with time” period finally comes to an end and a longer than normal period of subsiding mantle is waiting there for it, the Plio-Pleistocene mountain building period that occurred around the planet would be the result.
29<18.3 These illustrations below represent a combined continental spreading center arrangement similar to that of the Atlantic’s. The oceanic crusts are attached to their parental continents as they have been since they first divided.
29<18.52 In the image at left the individual eruption tracks of the Yellowstone Super volcano show the periodic movement of the North American continent as it is forced west over the remnants of the Farallon and Pacific plates by the actions of the Atlantic oceanic plates described above as they unload their inventory of GPE derived compression.
29<18.53 Each date represents in millions of years, the exact time period that the continental plate slowed or even possibly became almost stationary, and was then subsequently followed with the "Mantle thermal pulses" reactivating the volcano hotspot/mantle-tear to erupt again through the overriding plate. But eventually the "Mantle thermal pulse" would end and the mantle subsides once more and begins loading those Atlantic oceanic plates above with tremendous levels of compression that would eventually drive the North American plate to its next position. This would repeat with each mantle thermal pulse as is show by each individual eruption's position.
29<18.54 A very important point to make here is there is currently not one hypothesis available to explain how the North American continent is driven over the Farallon and Pacific plates. There is no down going slab pulling it or any way a mantle convection current could directly engage it.
And we must not forget this paper;
http://www.dst.uniroma1.it/sites/default/files/doglioni/E6-15-03-13-TXT.aspx.html
MANTLE DYNAMICS AND PLATE KINEMATICS
Carlo Doglioni, La Sapienza University, Rome, Italy
Roberto Sabadini, University of Milan, Italy
29<18.56 ". . . . . none of the proposed models of mantle convection can account for the simpler pattern in plate motion we observe at the surface, nor has a unique solution been proposed for how material in the mantle convects. At the moment there is no way to link mantle dynamics and plate kinematics at the surface, considering that the mantle and lithosphere are detached. The Atlantic and Indian ridges are in fact moving apart with respect to Africa, proving not to be fixed both relative to each other and relative to any fixed point in the mantle. This evidence confirms that ocean ridges are decoupled from the underlying mantle."
29<18.57 What would we expect to see during those mountain building periods?
29<18.58 The GPE is greater during these periods and would compress the Atlantic oceanic plates to greater levels. It would actually raise the oceanic plate’s elevation, crushing the boundary substantially over time.
29<18.59 The boundaries newest infill would be the thinnest and softest material. The greater levels of GPE would crush these weakest areas of the crust, eventually transforming this boundary into the largest oceanic mountain range on the planet.
29>18.6 Extraordinary levels of divergent boundary infill during extended periods of outward mantle displacement (below) is crucial for the mountain building periods to occur.
29<18.7 This material is what will provide the higher levels of gravitational potential energy derived compression that will later be needed when the mantle subsides to drive the planet’s crust into kinetic movement beyond the subduction trenches rate of resistance and redirect the divergent GPE to an alternate kinetic path of least resistance in the form of mountain ranges like the Himalayas and the Andes.
29>18.71 The prior period in question would be when the Basin and Range Province was created along with many other documented cases of crustal extension that took place in the Miocene.
29<18.8 This model proposes that the mantle’s outward movement at these times opens all of the divergent boundaries to magma intrusion simultaneously. This periodic boundary infill material is what will later produce the increase of gravitational potential energy in the crust that drives subduction. And in the case of the Mid Atlantic Ridge, this material will produce, when the mantle subsides, the forces needed to drive the various subducted plates under the two opposing continents, and too, for example, the energy to drive N. America over the Farallon and Pacific plates.
29<18.81 When the thermal cycle subsequently changed direction and the mantle moved downward, the opposing continents of the Atlantic Ocean moved down also, converting their gravitational potential energy into kinetic that begin compressing the oceanic plates of the Atlantic.
29<19.0 These illustrations below represent a combined continental spreading center arrangement similar to that of the Atlantic’s. The oceanic crusts are attached to their parental continents as they have been since they first divided.
29<19.1 (1) The period of thermal expansion has ended and the mantle has begun to subside. As the Earth’s entire crust moves downward the two continents pictured will be forced towards each other.
29<19.2 (2) The spreading center will close and compression in proportion to the continent’s movement will begin to deform and raise the boundary area. The thermal cycle’s mountain building compressional periods are only 4 +/- million years long, so the oceanic crust and boundary only need to endure the growing GPE until the cycle changes once again to thermal expansion.
29<19.3 (3) and (4) The entire oceanic crust must rise up as the boundary area becomes less compressible, the older and thicker crust further back from the boundary can resist the energies more easily without expressing the vertical displacement taking place at the boundary.
29<19.1 This model can be used to decipher some rather new and extrodinary revelations about the Atlantic MOR.
29<19.2 Due to uneven compressional loading of the oceanic plates some of the narrower sections will need to process a much larger portion of GPE. And due to this substantially larger loading of the narrower and much smaller plate widths these sections will eventually fail and slip vertically along their transitional faults.
Images and cross sections above and those below were furnished through and in no way endorsed by http://www.geomapapp.org using Global Multi-Resolution Topography (GMRT) Synthesis,
Ryan, W. B. F., S.M. Carbotte, J. Coplan, S. O'Hara, A. Melkonian, R. Arko, R.A. Weissel, V. Ferrini, A. Goodwillie, F. Nitsche, J. Bonczkowski, and R. Zemsky (2009), Global Multi-Resolution Topography (GMRT) synthesis data set, Geochem. Geophys. Geosyst., 10, Q03014, doi:10.1029/2008GC002332.
Data doi: 10.1594/IEDA.0001000, through http://creativecommons.org/licenses/by-nc-sa/3.0/us/
29<19.21 The crust in these higher compressional energy fault sections are located at the narrowest point of the Atlantic basin in the equatorial region. This model will show these areas will need to move upwards, higher than the much wider sections to the north and south, to relieve the higher compressive stresses that they are exposed to.
29<19.22 And when there is a large differential between the quantity of divergent boundary material and the degree of mantle subsidence, as is the case with the Pleo-Pleistocene, there would be a tremendous amount of compression in the crust that would produce energies beyond what any convergent boundary could process. The Himalayas, the Andes and all other convergent and divergent boundaries are where this energy became stored. And in the case of the Atlantic ridge the energy level would be more than that divergent boundary could hold.
29<19.23 If an oceanic divergent boundary was located very close to the center of the ocean basin, as is the case of the Atlantic, with the oceanic crust extended unbroken from each continent, the compressive energy could be expected to have likely raised the oceanic crust’s center in a wide arch.
29<19.24 And it would remain raised until the GPE in the crust would be slowly removed as the North American continent was pushed over the Pacific and Farallon plates.
29<19.3 But this boundary arrangement has a built in vulnerability. The boundary alignment would probably include the likelihood that the divergent boundary materials would begin to fail before all of the GPE could be removed through the movement of the opposing continents and would result in an arrangement resembling the current Atlantic ridge.
29<19.31 As the GPE began to wane the compression that kept the boundary raised would likely allow the crust sections on either side of the ridge to sag and this would crush the lower edges of the divergent boundary together, deforming them and moving the boundary upwards.
29<19.4
http://www.researchgate.net/publication/221939638_The_Vema_Transverse_Ridge_(Central_Atlantic)
29<19.41 "Multibeam morphobathymetric coverage of the entire Vema Transverse ridge shows it is an elongated (300 km), narrow (<30 km at the base) relief that constitutes a topographic anomaly rising up to 4 km above the predicted thermal contraction level. Morphology and lithology suggest that the Vema Transverse ridge is an uplifted sliver of oceanic lithosphere. Topographic and lithological asymmetries indicate that the transverse ridge was formed by flexure of a lithospheric sliver, uncoupled on its northern side by the transform fault. The transverse ridge can be subdivided in segments bound by topographic discontinuities that are probably fault-controlled, suggesting some differential uplift and/or tilting of the different segments. Two of the segments are capped by shallow water carbonate platforms, that formed about 3–4 m.y. ago, at which time the crust of the transverse ridge was close to sea level. Sampling by submersible and dredging indicates that a relatively undisturbed section of oceanic lithosphere is exposed on the northern slope of the transverse ridge. Preliminary studies of mantle-derived ultramafic rocks from this section suggest temporal variations in mantle composition". . . . . .
29<19.42 "Studies of depth-sensitive fossils and diagenetic textures in the dredged lime-stones have provided the rough outlines of a paleodepth versus age history for the transverse ridge crest. The summit of the transverse ridge was at or above sea level up to about 3 m.y. age, and has since subsided to its present depth (Bonatti et al., 1983')". . . . . . .
29<19.43 "We carried out one dredge on the limestone cap (dredge EW9305-1), recovering a large block of coral resting on a platform of coarse cemented biogeneous calcareous sand, plus smaller pieces of heterogeneous cemented biogeneous limestones, and half a dozen rounded pebbles resembling beach pebbles. . . . . .These new samples are compatible with the previous interpretation that the limestone cap was formed near sea level and subsided to its present location."
29<19.44 "This is a horizontal, prominent reflector, which has been interpreted as an erosional surface, lying within the limestone platform, which was planned off at sea level when the transverse ridge stood higher than at present."
29<19.51 The evidence of mantle oscillation on 3-4 million year periodicities allow us to locate other periods of extreme crustal compression within this same boundary area.
29<19.6 http://www.researchgate.net/profile/Luca_Gasperini/publication/221939595_Lower_Cretaceous_to_Eocene_sedimentary_transverse_ridge_at_the_Romanche_Fracture_Zone_and_the_opening_of_the_equatorial_Atlantic/links/02bfe50e54bab7655e000000.pdf
29<19.61 "The Romanche transform offsets the Mid Atlantic Ridge by about 900 km and is the largest of a set of major equatorial Atlantic fracture zones. Assuming for the crust adjacent to the transform an average spreading rate of 1.75 cm/yr (Cande et al., 1988) and a constant ridge/ transform geometry, the age offset of the Romanche transform is roughly 50 million years. A transform-parallel transverse ridge, running adjacent to the northern side of the fracture zone is particularly prominent for a stretch of several hundred kilometers centered opposite to the eastern ridge/ transform intersection (RTI), where the topographic anomaly reaches 4 km above the predicted thermal contraction level (Bonatti et al.,1994). Seismic reflection data and extensive rock sampling indicate that the western portion of the transverse ridge . . . . consists of slivers of uplifted oceanic crust and upper mantle (Bonatti et al.,1994). The summit of the transverse ridge is capped in this area by Miocene shallow water limestones that reached above sea level about 20 ma, and then subsided at a rate faster than that of “normal” lithospheric cooling. (Gasperini et al.,1997a). . . . .The presence of such a thick sedimentary sequence, including deep-water sediments as old as 140 Ma, less than 150 km from the RTI, does not fit a normal sea-floor spreading scenario and opening of the equatorial Atlantic."
29<19.62 Another prediction that this model can make is the crust in these higher compressional energy fault sections near the equatorial regions will need to move upwards, higher than the much wider sections to the north and south, to relieve the higher compressive stresses that they are exposed to in these narrower areas.
29<19.9 This model explains that the Vema and Romanche ridges, located in the central Atlantic and being so close to each other, is not random or a coincidence. If the mantle was to move down ever so slightly and move all the plates towards each other simultaneously, the four opposing continents of the Atlantic would compress in the manner seen below;
29<20.01 The narrower section would process this disproportionate and higher continental-movement-to-basin-width-ratio by either compressing the divergent boundary more or raising it higher than the adjacent and wider basin areas to the north and the south were raised.
29<20.1 Almost 20 million years earlier than the Pleo - Pleistocene era a similar period of extreme crustal compression raised a narrow section of crust above sea level within that same narrow equatorial corridor that the Vema Transverse Ridge resides. It would thus seem likely that the entire Atlantic crustal area was compressed in unison, allowing the Vema and Romanche ridges to attain the highest points in reference to depth.
29<20.2 This narrow section of the Atlantic crust is required to accommodate the greater latitudinal metric generated by the crustal subsidence that is produced at the equator. The highest amount of surface compression that the crust can generate is realized by the 40,008 km (24,859.82 miles) of the equator's circumference. This will result in this narrow section of oceanic crust to rise to greater heights than the wider expanses of seafloor that lie to the north and south of this area.
29<20.21 We have seen with the evidence shown above that the Atlantic seafloor;
"show(s) oscillations of ,3–4 Myr superimposed on a longer-term steady increase with time. The time lag between oscillations of mantle melting and crustal thickness indicates that the solid mantle is upwelling at an average rate of ,25mmyr, but this appears to vary through time."
29<20.3 We have also seen how well those oscillations fit the periodic plate tectonic movement that is seen by the Yellowstone caldera's movement across N. America.
https://geoconvention.com/wp-content/uploads/abstracts/2013/183_GC2013_Episodic_Tectonics.pdf
Episodic Tectonics in the Phanerozoic Succession of the North American Arctic and the “10 Million Year Flood”
Ashton Embry, Geological Survey of Canada, Calgary, aembry@nrcan.gc.ca
Benoit Beauchamp, University of Calgary, Calgary, bbeaucha@ucalgary.ca
Keith Dewing, Geological Survey of Canada, Calgary, kdewing@nrcan.gc.ca
James Dixon, Geological Survey of Canada, Calgary, jdixon@nrcan.gc.ca
Conclusions
29<20.41 “Fifty-six, large magnitude sequence boundaries have been delineated in the Phanerozoic succession of Arctic North America. The characteristics of the boundaries indicate that they were primarily generated by tectonics. The boundaries occur with an approximate 10 million year frequency (9.8 +/- 3.1). Each boundary was generated during a tectonic episode interpreted to reflect a mantle-driven, plate tectonic reorganization and consequent changes in regional stress fields. Such episodes likely lasted for a few million years and were separated by longer intervals of relative tectonic quiescence. There are indications that the recognized tectonic episodes affected basins throughout the world.”
29<20.5
http://assets.geoexpro.com/legacy-files/articles/Sequence%20Stratigraphy%20Meets%20Plate%20Tectonics.pdf
29<20.51 "Thus I was faced with the hard-toescape interpretation that significant tectonic episodes affected large areas of the Triassic world and that they were separated by long intervals of relative quiescence. Derek Ager’s oft quoted quip that "the stratigraphic record is like a soldier’s life; long intervals of boredom separated by short intervals of terror" took on new meaning. The Triassic was of course the time when Pangea was in full bloom and one way of rationalizing such widespread tectonics was that I was actually looking at only one continent, albeit a super one. I then looked at my Jurassic unconformities that also had all the indicators of a tectonic origin." - Ashton Embry
29<20.6
https://geoconvention.com/wp-content/uploads/abstracts/2013/183_GC2013_Episodic_Tectonics.pdf
29<20.61 "A given tectonic episode began with the initial uplift of the basin margin (start of base level fall) and ended with the collapse and marine flooding of the margin (maximum flooding surface). The sequence boundary was generated during the tectonic episode and represents the time of maximum uplift and basinward extent of the unconformity. It is estimated that the duration of each of the tectonic episodes was in the range of a few million years and was significantly shorter than the intervening times of tectonic quiescence."
29<20.7 We can now imagine that as the mantle eventually subsided, the continents, who's oceanic plates form the world's oceanic basins, would have been moved towards each other and compressed. (images below) This compression would have caused the basin margins to be forced upwards, as was observed by Embry et al above. And as the compression continued to grow the entire oceanic basin rose. As this happens it would have eventually caused the Mid Ocean Ridges to fail and rupture vertically in the manner shown in the preceding section. This would have caused the coastal margin to lower and produced those "Fifty-six, large magnitude sequence boundaries" that according to the above research have been observed around the world. Then the continents eventually moved apart to a lower energy state from the immense strain of the GPE produced by the raised oceanic crust.
29<20.81
http://assets.geoexpro.com/legacy-files/articles/Sequence%20Stratigraphy%20Meets%20Plate%20Tectonics.pdf
29<20.9 These two separately derived scenarios have very similar descriptions of oceanic crustal stresses and dynamics. The fact that the timing and duration of these events are of similar metrics would seem to be beyond simple coincidence, when you include the examples of the Yellowstone complex, the Himalayas and the Andes record of similar timing and duration, it would seem there is more than a casual connection at work here.
29<21.0
https://geoconvention.com/wp-content/uploads/abstracts/2013/183_GC2013_Episodic_Tectonics.pdf
29<21.02 In this paragraph above we can see that this model is well defined in their observations of the episodic timing, plate movement, and most importantly, the correlation " with significant carbon isotope excursions" This model's thermal fingerprint of the strain energy thermal content, that has repeatedly been shown to occur within the solar magnetic and seismic histories presented in this paper, is once again where we would expect it to be.
29<21.03
https://www.sciencedirect.com/science/article/pii/S1674987120301444#bib44
A 32-million year cycle detected in sea-level fluctuations over the last 545
Abstract
29<21.04 "Spectral analyses of past relative sea-level oscillations as represented by the ages of 57 Phanerozoic (the last 545 Myr) stratigraphic sequence boundaries from the Canadian Arctic show a strong spectral peak at 32 Myr (>99.9% confidence). These findings concur with previous reports of significant cycles with periods of around 30 Myr in various records of fluctuations of sea level, and in potentially related episodes of tectonism, volcanism, climate, and biotic extinctions. Sequence boundaries commonly coincide with stage boundaries based on biostratigraphy, and are correlated with episodes of extinction and times of flood-basalt volcanism. The connection between tectonics and sea-level variations may come from changes in rates of ocean-floor spreading and subduction, intraplate stresses from plate-reorganizations, and pulsations of hotspot volcanism. These coordinated periodic fluctuations in tectonics, sea level and climate may be modulated by cyclical activity in the Earth’s mantle, although some pacing by astronomical cycles is suspected."
29<21.05 Well, that abstract above hints at, if not actually verifies, every interrelated mechanism this model has been showing and describing in a reasonable, if not rigorous detail since 2015.
29<21.07 "Assuming for the crust adjacent to the transform an average spreading rate of 1.75 cm/yr (Cande et al., 1988) and a constant ridge/ transform geometry, the age offset of the Romanche transform is roughly 50 million years."
29<21.08 "The presence of such a thick sedimentary sequence, including deep-water sediments as old as 140 Ma, less than 150 km from the RTI, does not fit a normal sea-floor spreading scenario and opening of the equatorial Atlantic."
29<21.10 This model can not only predict these very specific events, but can also give very specific details of how they are intimately tied to not only the mantle and its mantle thermal pulses, but also the forced climate transition that took place leading up to, during, and after these events took place.
29<21.11 As shown previously on this page, we showed a very long and active period of "mantle thermal pulses" that accumulated an extraordinary quantity of divergent boundary materials that in millions of years later produced extraordinary levels of compressive energy in the oceanic plate that in turn produced phenomena such the “Fifty-six, large magnitude sequence boundaries" seen in the sedimentary record.
29<21.12 But the model also predicts that the period of higher divergent boundary activity will correlate to warmer climate periods that will then change to substantially cool climate periods as the divergent boundaries lower their activity, while the oceanic crustal plates try to dispose of their GPE into their attached continental margins that are displaced vertically to accommodate the growing GPE that is compressing the boundaries and producing massive flooding of the margin areas.
29<21.13 These are very specific predictions. A very warm period lasting tens of millions of years that precedes a period where substantial sedimentary deposits on continental margins above and below sea level are removed from the geologic record and the climate becomes substantially cooler for an extended period of tens of millions of years.
29<21.14 In the recent research paper below this exact scenario is discussed.
29<21.15
https://phys.org/news/2024-10-professor-global-gap-geologic.amp
"". . . . To his surprise, he instead found—nothing."
29<21.17 The journal Earth-Science Reviews has published Burton's findings, which reveal the existence of a global unconformity—or gap in the rock record—around the edges of every continent at the time of the pivotal greenhouse-to-icehouse climatic transition.
29<21.18 In addition to challenging conceptual models widely used for the past half-century about the relationships between sea levels and sediment movement in the deep oceans, Burton said his discovery has left him and his co-authors wondering: Where in the world did all the sediment go?
29<21.19 "This is something we weren't at all expecting to discover," said Burton, who recently joined the faculty of MSU's Department of Earth Sciences in the College of Letters in Science. "We'd set out to find lots of deposition, lots of sand. Instead, we're finding a gap in the rock record. That sediment is missing.""
29<21.20
https://www.sciencedirect.com/science/article/abs/pii/S0012825224002393?via%3Dihub
Global Eocene-Oligocene unconformity in clastic sedimentary basins
Zachary F.M. Burton, Tim R. McHargue, Stephan A. Graham
Author links open overlay panel
29<21.21
Abstract
. . . . . "We choose to focus on the Eocene greenhouse to Oligocene icehouse transition, a period that is remarkable as the most profound climatic transition of the Cenozoic, and a period characterized by extreme cooling and expansion of polar ice, sea-level fall, and global changes to ocean circulation. We perform a comprehensive review of marine siliciclastic basin literature to produce a global inventory of Eocene-Oligocene unconformities. We find evidence for a prominent Eocene-Oligocene unconformity in sedimentary basins along the margins of every continent. The globally-widespread distribution of unconformities suggests global controls on their development. Furthermore, Eocene-Oligocene erosion surfaces are common in shelf settings, as well as in deep-water settings from slope to basin floor, indicating unconformity-generating processes across a wide range of water depths. Global sea-level fall may have driven subaerial shelf exposure and erosive down-slope processes including submarine canyon cutting and mass wasting. Meanwhile, the intensification of deep-ocean thermohaline currents potentially induced erosion of not only pelagic successions, but also of clastic successions. Overall, our documentation of globally-widespread Eocene-Oligocene unconformities suggests global controls, of which the extreme climatic and oceanographic changes of the greenhouse to icehouse transition seem particularly compelling."
29<21.22
Discussion: mechanisms for Eocene-Oligocene unconformity formation
"The prevalence of widespread Eocene-Oligocene unconformities in marine siliciclastic successions of sedimentary basins along all continental margins inherently requires global mechanistic explanations . . . "
29<21.23 The operation of the plate tectonic mechanism of this model predicts the results that are mentioned and being studied in the paper above.
-----------------------------------------------------------------------------------------------------------------
Page 29
Correlation of
Extensional and Compressional Episodes to
Miocene, Pliocene and Pleistocene Temperature Record.
------------------------------------------------------------------------------------------------------------------------------------------------------------------------------------------------------------------
29<21.24 The model contends that the current divergent boundary metrics are the result of a strain energy response to the core/outer core's thermal expansion.
29<21.25 The mantle is 2,900 kilometers (1,800 mi) thick, that constitutes about 84% of Earth's volume. The Earth is 40075.16 kilometers in circumference with divergent boundary metrics measured in millimeters per year. The Pacific mid ocean ridge is the largest at 80-120 mm per year and the North Atlantic being 25 mm per year.
29<21.26 The total lateral displacement of the Basin and Range extension varied from 60 – 300 km over a period of around 7+/- million years. Just 42 mm a year of displacement at the Basin and Range area in that 7 million years time from around 17 to around 10 million years ago from the early to middle Miocene will give you the 300 km of displacement. Seems a reasonable figure compared to current Pacific at 80-120 mm per year and the North Atlantic being 25 mm per year.
29<21.27 The energy in molecular level thermal expansion is immense. But the thermal expansion energy displacing the divergent plate boundaries is not taking place in the mantle. The mantle is being outwardly displaced by the core's thermal expansion due to the increase of current and field by induction. The thermal expansion of the core's liquid iron will move outward with no regard to the mass of the mantle trying to compress it. It will not be stopped. The thermal expansion energy of core is multiplied by the thickness of the mantle similar to the inverse square law.
29<21.28 This study below shows an extensional event in Antarctica, also during the Miocene, but of course suggests the current standard model's reason, mantle upwelling in regards to a plume as a cause.
http://geodynamics.u...nts/frbdm08.pdf
29<21.30 "Our study documents two subsequent episodes of deformation occurring from Middle Miocene onward, concurrently with the McMurdo volcanism in the Admiralty Mountains region. The first is dextral transtensional whereas the second is purely extensional."
29<21.4 And of course this model suggests the strain energy derived thermal content enters the ocean through the 80,000 km (49,700 mi) long Mid-Ocean ridge system and is then delivered by the thermohaline circulation of the world ocean.
29<21.41 This NASA article about Antarctica warming in the Miocene expresses this nicely.
29<21.42
29<21.4 "Scientists began to suspect that high-latitude temperatures during the middle Miocene epoch were warmer than previously believed "
29<21.5 "The climate was suitable to support substantial vegetation -- including stunted trees -- along the edges of the frozen continent."
29<21.51 "Along the edges" where all that ocean thermohaline circulation heat is warming the atmosphere.
29<21.52 "the research team found summer temperatures along the Antarctic coast 15 to 20 million years ago were 20 degrees Fahrenheit (11 degrees Celsius) warmer than today, with temperatures reaching as high as 45 degrees Fahrenheit (7 degrees Celsius). Precipitation levels also were found to be several times higher than today."
29<21.53 "The peak of this Antarctic greening occurred during the middle Miocene period, between 16.4 and 15.7 million years ago."
29<21.6 And this extensional event; http://www.earth-prints.org/bitstream/2122/1511/1/01%20mascle.pdf
4. Conclusions
"Most of the structural characteristics from both features indicate important changes of tectonic styles around the Miocene. Thus, in the Mediterranean, the Tortonian to Messinian time span appears (to) be a key period, marked first by a wide spread extension episode, superimposed on previous collisional features both within the Tyrrhenian and Aegean domains."
29<21.7 So there appears to be a correlation between surface extensional events and warmer climate periods. And as I have shown there is ample evidence of a correlation to the mountain building period of the Himalayas and the cooling climate.
29<21.71
ANNALS OF GEOPHYSICS, SUPPLEMENT TO VOL. 49, N. 1, 2006
Mountain uplift and the Neotectonic Period
CLIFF D. OLLIER
School of Earth and Geographical Sciences, University of Western Australia, Perth, Australia
29<21.8 This graph below shows this cooling, starting at the end of the Miocene (not shown) beginning at the left side of the top graph, and continuing through the mountain building period;
29<21.9 By the end of the Miocene the core temp began to cool and the mantle slowly contracted and began reloading the crust's inventory of compression, a long period of subduction was underway. As the crust is increasingly loaded with stored compression from the contracting mantle due to the resistance in the subduction trenches, the crust begins a period of global mountain building that occurred during the "Early and Middle Pleistocene." The compression continued to bleed off into the trenches until the crust began a slow and low energy period of activity. It's interesting to think the solar magnetic energy cycle that extended the Basin and Range was stored as mass in the crust then converted by gravity to kinetic energy that then lifted the mass of the Himalayas millions + years later.
-----------------------------------------------------------------------------------------------------------------
Page 30
Model for
Mountain Ranges on Continental Interiors
-----------------------------------------------------------------------------------------------------------------------------------------------------------------------------------------------------------------
30<22.0 The "Plate tectonics has no plausible explanation for mountains on passive margins or continental interiors" statement can also be answered by the expansion - contraction cycle of the mantle. Take the North American Cordillera (Rocky Mountains) for example, a long period of contraction of the mantle after a similarly long period of expansion with extension and thinning of the lithosphere, could provide the planation necessary to produce these structures. It’s that laminated structural dynamics again, the lithospheric layer is stretched and the overlying continental rock is extended and tensioned causing thousands of parallel faults during the long expansion period. Over the long period of expansion the surface erosion processes continually move the fractured debris downward into the continuously lowering and extending crust. The stretched, fractured and eroded material will have nowhere to go but up during the long contraction (shortening) that follows.
30<22.1 With the Rocky Mountains, the continent fractured in multiple parallel faults from high thermal expansion, while the lithosphere stretched and thinned. When the contraction period was in full compression the lithosphere compressed and folded under the fractured sections which were ruptured at high angles that bear witness to the large lateral expansion and then contraction that this region underwent.
30<22.2 Picture below was taken by Jesse Varner and was modified by Aza Toth both of whom have no connection with this author or this paper.
30<22.3 The Flatirons Mountains of the North American Cordillera (Rocky Mountains) outside of Boulder Colorado shows the results of being contracted over compressed and folded lithosphere forming a tilted assemblage of overlapping and stacked slabs of crust. These structures were eroded out over 50 + million years. These are large thermal cycle events involving a core temperature variation over millions of years. This is how mountains are built in continental interiors.
-------------------------------------------------------------------------------------------------------------------------------------------------------------------------
Page 31
A Model for
Plate Dynamics of
Oceanic Slab Break-off and Relocation
------------------------------------------------------------------------------------------------------------------------------------------------------------------------------------------------------------------
31<22.4 The Coast Range along the Pacific coast from the Baja Peninsula to Canada is a likely product of expansion-contraction cycles. As the mantle expanded, the ocean crust became tensioned pulling on the western coastal area. The Pacific plate's massive expanse gave it a high demand for the differential of allowable movement, 80-120 mm per year currently and probably greater during the Basin and Range extension of which time I believe the Pacific coast was extended. The coast of the northwest had overrode the last remnant of the subducted Farallon Plate, which at this time 10 to 17 million years ago had been followed by the East Pacific Rise's divergent boundary. The allowable movement in the Pacific Plate during the thermal expansion cycle produced a shorter but proportionate extension of the coastal plain area and may have lowered the basins of Puget Sound and Willamette Valley.
31<22.5 This scenario also includes the San Andreas Fault that was originally at one time the result of the shear tension fracture of the overran and held Pacific Plate. The fault can be viewed at various road cuts exhibiting the anti-intuitive form of the Pacific plate overlaying the North American plate, the exposed fault line cutting diagonally across the exposure. The current theory suggests the fault has been raised from great depth by uplift and then exposed thru erosion.
31<22.6
http://earthquakes.ou.edu/Wilson_Tejon_Pass.pdf
31<22.7 "rate of uplift being estimated as 2-5 millimeters per year based on plate motion considerations and modeling (Smith and Sandwell, 2003), as well as age determination analyses (Spotila et al., 2001). These rates indicate very intense exhumation of more than 5 kilometers (3.1 miles) since the Pliocene, which would likely expose deep portions of the fault-zone and gouge material and are consistent with estimates of nearby exhumed fault zones (Evans and Chester, 1995)."
Images A,B,B1,B2,C,C1,D,E,G,I,J used and modified by this author in the following section were furnished through and in no way endorsed by http://www.geomapapp.org using Global Multi-Resolution Topography (GMRT) Synthesis,
Ryan, W. B. F., S.M. Carbotte, J. Coplan, S. O'Hara, A. Melkonian, R. Arko, R.A. Weissel, V. Ferrini, A. Goodwillie, F. Nitsche, J. Bonczkowski, and R. Zemsky (2009), Global Multi-Resolution Topography (GMRT) synthesis data set, Geochem. Geophys. Geosyst., 10, Q03014, doi:10.1029/2008GC002332.Data doi: 10.1594/IEDA.0001000, through http://creativecommons.org/licenses/by-nc-sa/3.0/us/
31<22.8 Image A.
31<22.9 The Basin and Range extensional episode supplied a means to understand the likely position and depth of the already subducted Farallon Plate, and the Pacific Plate that followed it under the western continental crust in an almost parallel horizontal trajectory with the surface.
31<22.10 This section being the most recently produced had the lowest density with the highest temperature and that made it both too buoyant to sink and too soft for compression driven subduction into the deeper region of the asthenosphere. So once it was over ridden by North America, the Pacific plate stayed at a shallow almost straight inline path into the transitional area of the continent.
31<22.11 And as it was shown prior in the research paper that describes the sudden and forceful conversion of a divergent boundary into a convergent boundary, thus leading to the likely conclusion that it is increasingly apparent that the subducting plate's path is simply determined by the path of least resistance. That the subducting oceanic plate will only go where the surrounding geology will allow.
31<22.12 Taking the above into consideration when evaluating the behavior of the Farallon and then Pacific plate's subduction under the N. American Continent, the shallow subduction idea fits the observations quite well. This model proposes that with every cycle the Pacific plate moved in tandem with the decoupled divergent boundary as they were slowly overran by the periodic movement of the North American plate. The compression driven westward movement of the overriding North American plate produced a compounded drag effect on the top surface of the subducted ocean plate. The friction produced an upward curl or pull back in the plate, balancing the downward force of gravity against the overriding plates frictional drag. This allowed the Farallon plate to extend at a flat trajectory well into the basement of the Basin and Range area of Western Nevada and possibly as far as Utah while simultaneously raising the overriding Basin and Range region.
http://en.wikipedia.org/wiki/Basin_and_Range_Province
The tectonic activity responsible for the extension in the Basin and Range is a complex and controversial issue among the geoscience community. The most accepted hypothesis suggests that crustal shearing associated with the San Andreas Fault caused spontaneous extensional faulting similar to that seen in the Great Basin. However, plate movement alone does not account for the high elevation of the Basin and Range region.
31<22.14 This embedment can only occur when there is periodic supplies of compression available in the crust, driving the Farallon Ocean plate from the west and the continental crust from the east. This subduction accounts for the substantial rise in elevation of the Basin and Range that resulted as the oceanic plate was subducting and vertically displacing the continental crust above it.
31<22.15 The Pacific Plate had followed the East Pacific Rise in under the continent, the cooling mantle's contraction giving the overlapping plates the massive kinetic energy that can only be provided by sources of these scales. The Pacific Plate in that latitudinal section was held by the Mariana Trench in the western Pacific and the overriding North American continent to the east. During one of the expansion cycles, while the Basin and Range extension was occurring (area C below), and while the overlying continental crust was being pulled by the now retracting ocean crust, the underlying oceanic lithosphere was breaking the overlying surface area into hundreds of parallel faults.
31<22.16
,http://earthquakes.ou.edu/Wilson_Tejon_Pass.pdf
"A reconnaissance survey (Brune, 2001) of the apparent gouge-zones at more than ten sites along the San Andreas fault between San Bernardino and Tejon Pass resulted in the following observations: 1) there are no obvious shear planes or bands parallel to the fault; 2) at distances up to 200 meters from the fault the gneissic or granitic structure appears to be undisturbed, in particular – unsheared, while it consists almost entirely of fine-grained powder, suggesting that the rocks were powdered without shear distortion; 3) the powder occurs only in gneisses and granites, not in shallow sedimentary rocks, and 4) the rock powder contains myriads of fine-scale conjugate shear bands with displacement consistent with a maximum principal stress generally normal to the San Andreas fault." "The dominant rock in the San Andreas fault-zone at Tejon Pass is a light color granitic rock body that to the casual observer appears as intact granite with a granitic texture and sub-gneissic foliation in some places. However, this granite is pervasively shattered into powder, has no apparent cohesion and has easily eroded into badlands topography along the outcrop"
31<22.17 Is this powdered rock an indication that the fracture was due to extreme tension fracturing and not shear stress? If the oceanic plate was overran by the continental plate and held during a thermal expansion (displacement) period, a catastrophic amount of tension would build, resulting in an explosive release. Tension stretches and thins any material if enough energy is applied; the stresses are to the microscopic level in the granite. There would be enough tensile stress derived energy released to cause large retraction of the plate in both sides of the fracture zone. This may be what caused the Sierra Nevada block to be lowered to the west and tilted, the west end dropping to the central valley elevation, while the east end of the block remained on the retracted ocean plate section that rebounded to the east. Current estimated time period of the westward tilting of the block has the beginning starting at 10 million years ago. The Sierra Nevada block now had a fulcrum by way of the broken upper edge of the eastern ocean plate remnant.
31<22.18
https://www.nps.gov/parkhistory/online_books/geology/publications/pp/160/sec2a.htm
31<22.19 ". . . . . .the present range consists essentially of a single massive block of the earth's crust that has been dislocated and tilted toward the southwest, apparently without suffering any appreciable bending or warping. (See fig. 1.) The Sierra Nevada is therefore properly termed a "block range." Even to the first geologists who explored the Sierra Nevada and the adjoining regions the real nature of its structure was apparent from its very shape and outlines. The long linear crest suggested the raised eastern edge of the block; the abrupt eastern escarpment, the side of the block exposed to view by the uptilting; and the gentle western slope, down which the main rivers flow, the slanting upper surface. The essential correctness of this early interpretation has been abundantly confirmed by later observers, who have collected considerable evidence, direct and indirect, showing that great master fractures extend along the eastern base of the range and around its curving southern part, and that on these master fractures the earth's crust has been dislocated, the Sierra block rising, and the block adjoining it on the east sinking, relative to each other, in the manner indicated by the arrows in Figure 1."
31<22.20
Is there evidence in the most recent research of an oceanic plate fracturing at these same massive scales in very similar circumstances?
31<22.21
This paper below describes such an event occurring at the Middle America Trench (MAT).
https://www.nature.com/articles/s41561-018-0229-y.epdf?referrer_access_token=HnO4WLMkwIDAFKrrT9rJIdRgN0jAjWel9jnR3ZoTv0PCVFi5Qgnv4AZa5anaERJuXVeI0Z9Swq0ea-YUFI2LVQ8XYXvH2evpIz7y0MpjjEksanddQ6LMf6qnf09O8vVzYYHDB5EnBhO9TcYikF3eacXtCXTB4no59PlmIpW7mzk464W0k1PzII2OrWtsMKJLpT438bM9HjQCsqWDqoP98Q%3D%3D&tracking_referrer=www.nationalgeographic.com
31<22.22
Deep embrittlement and complete rupture of the lithosphere during the Mw 8.2 Tehuantepec earthquake
Diego Melgar 1*, Angel Ruiz-Angulo 2, Emmanuel Soliman Garcia 3, Marina Manea 4,5, Vlad. C. Manea 4,5, Xiaohua Xu 6, M. Teresa Ramirez-Herrera 7, Jorge Zavala-Hidalgo 8, Jianghui Geng 9, Nestor Corona 10, Xyoli Pérez-Campos 11, Enrique Cabral-Cano 11 and Leonardo Ramirez-Guzmán 12
31<22.23 "The kinematic slip model of the earth quake challenges current structural models of subduction kinematics; there is normal-faulting (extensional) rupture throughout the entire lithosphere at a point where curvature is high and through portions that should be in flexural compression, and furthermore, rupture spreads well past the 650 °C geotherm (Figs. 1d and 4) into regions where global surveys find that no normal or thrust-faulting intraslab outer-rise-associated seismicity has ever been observed. At the nucleation depth in the bottom half of the oceanic lithosphere, the slab appears to be in tension, contrary to what is predicted from mere flexure"
31<22.24 "Combining these observations, we conclude that, in spite of slab curvature being high (Fig. 3a), the entire lithosphere is under wholesale deviatoric tension, explaining the unusual location of a normal-faulting mechanism in areas expected to be in compression and extending to the base of the lithosphere."
31<22.25 "Thus, combined seismological and geodetic observations of the 2017 Mw 8.2 Tehuantepec, Mexico, earthquake reveal that almost the entire oceanic lithosphere, including the mantle and crust, ruptured in a single large intraslab normal-fault earthquake. It is possible that the 1931 normal-faulting Mw 8.0 Oaxaca earthquake, just east of the Tehuantepec Ridge, similarly broke the entire lithosphere and that both events are part of an ongoing larger-scale breakdown of the slab; however, limited historical seismograms make that determination difficult. Here, we have shown conclusively, with a wide diversity of geophysical observations, that under the right conditions, large earthquakes can nucleate in and rupture through the entire oceanic plate, even for young subduction zones."
31<23.0 So, we now have additional observational evidence that supports this model's contention that oceanic slabs can be repeatedly exposed to tensional stresses that cause the complete breaking and sectioning of the subducted oceanic plates. And, that these exact processes were predicted and describe by this model years before these latest observations were made.
31<23.1 The observations made by the research paper above fit nicely with those made by Keenan et. al., https://www.pnas.org/content/113/47/E7359; where a newer divergent boundary spreading center was suddenly changed to a convergent boundary. We can now imagine that while this scenario in Keenan et. al. was transpiring, a convergent boundary plate section like the one mentioned above at the Middle America Trench, was initially and simultaneously breaking when that divergent boundary in Keenan et. al. was first developing. Then as the mantle began to subside, both boundaries rapidly changed to a convergent dynamic. The Keenan et. al. converging for the first time while the ones similar to the Middle America Trench were reestablished below and maybe even above the previously subducted plate materials.
31<23.2 This process then, would periodically provide a new layer of continental building material that will eventually break free and be later under laid by another section, and thus allow the oceanic plates that are subducting into convergent boundaries on continental margins to periodically fracture in a manner resembling what was observed at the Middle America Trench.
31<23.3 So, as we look at these continental margins we can see the results made by these periodic extensional energies. The complete rupture of the lithosphere during the Mw 8.2 Tehuantepec earthquake at the Middle America Trench occurred deep within the convergent boundary, while the San Andres is a specific example of what would happen when a specific oceanic plate section breaks free far under the edge of an overriding crust. The weight of the Sierra Nevada Batholith was probably a contributing factor to where this plate eventually fractured just below the batholith's western edge. And the Juan de fuca ridge just to the north is an example of what occurs when a similarly tensioned, subducted and overridden section of oceanic plate was suddenly exposed to the energies of an adjacent catastrophic plate failure, resulting in the plate's sudden tension fracture failure localized at what is a considerable distance from the subduction zone where the plate was being consumed under the continental margin and where prior to this event lesser examples of these energies had been gradually processed and dispersed.
31<23.4 In the image below you can see the zig-zag tension fracture configuration of the now referred to Juan de Fuca and Gorda ridges and the Blanco Fracture complex as it runs north from its abrupt beginning at the Mendocino Fracture Zone. If we examine these structures through the lens of mantle displacement cycles these structures easily appear to originate as tension releasing mechanisms. Then as the mantle began subsiding and the energies change from tension to compression the much more ancient convergent boundary on the continental edge becomes the central location of plate energy dispersal.
31<23.5 Each of these seemingly different geologic phenomena share the same origin in the periodic cycles of mantle displacement. And once again we can also see how this model provides a source for new continental building materials.
Images and cross sections above and those below were furnished through and in no way endorsed by http://www.geomapapp.org using Global Multi-Resolution Topography (GMRT) Synthesis,
Ryan, W. B. F., S.M. Carbotte, J. Coplan, S. O'Hara, A. Melkonian, R. Arko, R.A. Weissel, V. Ferrini, A. Goodwillie, F. Nitsche, J. Bonczkowski, and R. Zemsky (2009), Global Multi-Resolution Topography (GMRT) synthesis data set, Geochem. Geophys. Geosyst., 10, Q03014, doi:10.1029/2008GC002332.
Data doi: 10.1594/IEDA.0001000, through http://creativecommons.org/licenses/by-nc-sa/3.0/us/
-----------------------------------------------------------------------------------------------------------------
Page 32
Evidence of Periodic Extension/Compression Cycles in Oceanic Lithosphere
------------------------------------------------------------------------------------------------------------------------------------------------------------------------------------------------------------------
32<23.6 Image C1. These images are in a series of higher resolutions of approximately the same location of the Atlantic Mid-Ocean Ridge. The ridge shows the lack of sediment that younger sea floor should exhibit. If the Mendocino structure had formed earlier in the sea floor's history, it would have had more sedimentary cover. If it had formed near the N.A. Continent it would have been covered like all the shoreline sea floor, in a thick outflow of continental sediment that far out paces the subduction processes that will consume the deposits millions of years in the future.
32<23.7 Images above and below used and modified by this author was furnished through and in no way endorsed by://www.geomapapp.org using Global Multi-Resolution Topography (GMRT) Synthesis,
Ryan, W. B. F., S.M. Carbotte, J. Coplan, S. O'Hara, A. Melkonian, R. Arko, R.A. Weissel, V. Ferrini, A. Goodwillie, F. Nitsche, J. Bonczkowski, and R. Zemsky (2009), Global Multi-Resolution Topography (GMRT) synthesis data set, Geochem. Geophys. Geosyst., 10, Q03014, doi:10.1029/2008GC002332.
Data doi: 10.1594/IEDA.0001000, through http://creativecommons.org/licenses/by-nc-sa/3.0/us/
32<23.8 The lower degree of cover in the anomaly (A) above, allows the age of this structure to be reduced to the approximate age of the Basin and Range and more accurately to the period after the ocean plate fracture when the plate sections on either side of the Mendocino were initially offset by a substantial degree, leaving a relatively small gap in the fracture that was immediately filled by the hydraulic magma. This small infill became the first of many that were the result of the expansion-contraction cycle's mechanical advancement of the northern plate section and the lack thereof, of mechanical movement in the now disconnected southern (below the Mendocino) oceanic plate section. Something else worth mentioning, the Atlantic sea floor images show a finer grain in the ridge infill compared to the Mendocino anomaly. The two opposing continent's gravitational kinetic energies apply a tremendously larger amount of compression to the Atlantic divergent plate boundary infill. This would also explain the tremendous size and elevation of the Atlantic Mid-Ocean ridge system.
32<23.9 I have to be honest, the Google maps images are much more detailed for these seafloor examinations but you cannot use them. I suggest you take a look at them to see the improved resolution.
32<23.10 Image D. In this close-up of the Mendocino structure, the original edges (A) of the fracture are clearly seen. The infills of repeated thermal cycles are evident in the center (D). You can almost count how many of the expansion-contraction cycles have occurred. You can also see a single small intrusion (C) that may have broken loose during a cycle and then was lifted by new magma inflow. Once the mantle begins pressurizing the hydraulic magma as the crust is displaced, the magma will easily move into this mini divergent boundary as it slowly moves apart.
32<23.11 When the thermal cycle changes due to the cooling and contracting mantle the infill material will be compressed by the overwhelming forces of contraction that will form the new infill and surrounding seafloor to each other. You can see the deformation in the ocean plate on both sides of the anomaly (B). The continuous process started with a small infill; remarkably it is probably the one at the southern point (E). The anomaly has expanded approximately 1100 km. (F) since its beginning. The structure will continue to grow, slowly with each cycle, filling in and then moving to the west. This process will continue as the fracture and anomaly are over ran by the North American Continent and consumed. This is a strong record of the core-mantle thermal cycles.
32<23.12
Referring back to;
Mantle thermal pulses below the Mid-Atlantic Ridge and temporal variations in the formation of oceanic lithosphere
Enrico Bonatti*†‡, Marco Ligi*, Daniele Brunelli*†, Anna Cipriani‡, Paola Fabretti*, Valentina Ferrante*†, Luca Gasperini* & Luisa Ottolini§
* Istituto di Scienze Marine, Geologia Marina, CNR, Via Gobetti 101, 40129, Bologna, Italy
† Dipartimento di Scienze della Terra, Universita` “La Sapienza”, Piazzale Aldo Moro 5, 00187, Rome, Italy
‡ Department of Earth and Environmental Sciences, Lamont Doherty Earth Observatory, Columbia University, Palisades, New York 10964, USA
§ Istituto di Geoscienze e Georisorse, Sezione di Pavia, CNR, Via Ferrata 1, 27100, Pavia, Italy
32<23.13 "A 20-Myr record of creation of oceanic lithosphere at a segment of the central Mid-Atlantic-Ridge is exposed along an uplifted sliver of lithosphere. The degree of melting of the mantle that is upwelling below the ridge, estimated from the chemistry of the exposed mantle rocks, as well as crustal thickness inferred from gravity measurements, show oscillations of ,3–4 Myr superimposed on a longer-term steady increase with time. The time lag between oscillations of mantle melting and crustal thickness indicates that the solid mantle is upwelling at an average rate of ,25mmyr, but this appears to vary through time."
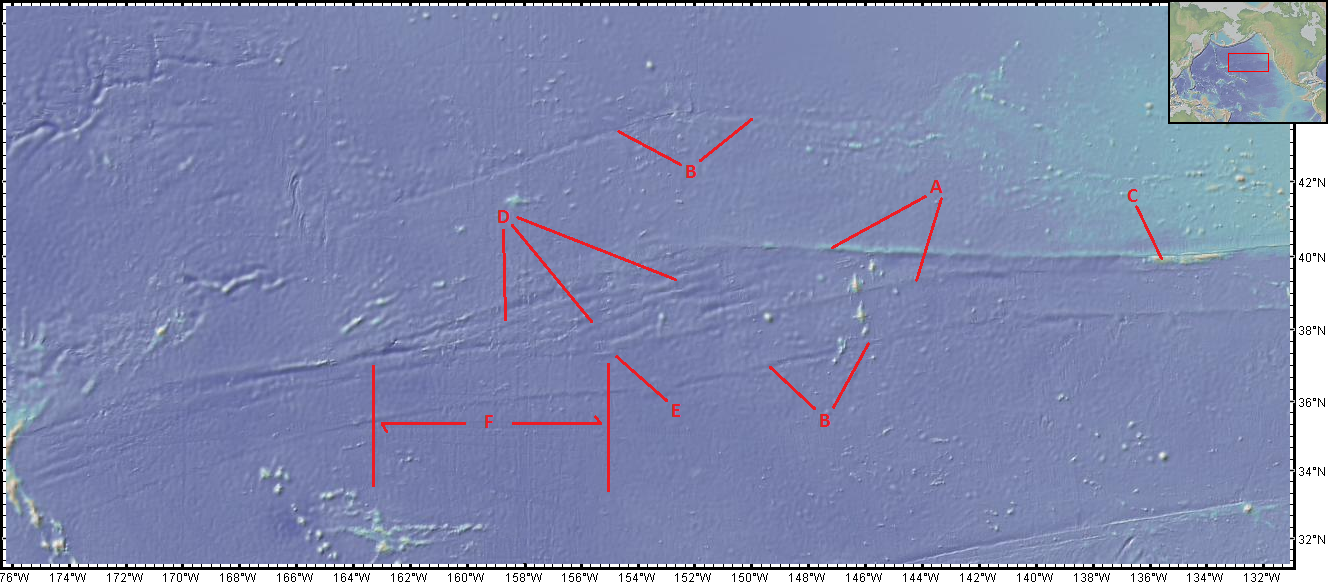
32<23.14 There are approximately 6-7 distinct cycle infills in the anomaly. At the 3-4 million year frequency rate of the paper above the first infill would date it back to around 18-21 million years, thus fitting nicely to the model's claim that it is concurrent with the formation of the Basin and Range and the San Andreas during the Miocene Epoch that began 23.03 million years ago.
32<23.15 Death Valley (black oval) lies at the southern end of a geological trough known as Walker Lane (outlined in red), which runs north into Oregon. The crust in the Death Valley region between Lake Mead and the southern Sierra Nevada has been extended by as much as 240 km (150 miles).
32<23.16 The floor of the valley is currently sinking 2.54 mm a year. The actual bed rock at the spreading zone lies below 3 miles of sedimentation. This spreading center is currently active indicating the mantle is expanding at this time. In the model an expanding mantle indicates an increasing core temperature.
32<23.17
Base image source: Shaded Digital Elevation Model Data of Nevada, NASA
32<23.18 A slice through the highest and lowest points in Death Valley National Park.
Image courtesy geomaps.wr.usgs.gov
-----------------------------------------------------------------------------------------------------------------
Page 33
A few images of Crustal Compression at Work
--------------------------------------------------------------------------------------------------------------------------------------------------------------------------
33<24.0 Image K. This section of oceanic crust looks like it has been under repeated periods of extremely high crustal compressive load. This is the path of least resistance at its best. The compressive load from a large ocean plate with the mass of the Pacific plate can overwhelm small weak sections of surface structure like this island archipelago that occupies the now 800 kilometer (500 mi) wide passage that extends between Cape Horn and Livingston Island which is part of South Shetlands archipelago that extends from Antarctica. South America is and has been moving west while the ocean floor section has been moving east.
33<24.1 Image K above was acquired from GeoMapApp at http://www.geomapapp.org using Ryan, W. B. F., et al. (2009), Global Multi-Resolution Topography synthesis, Geochem. Geophys. Geosyst., 10, Q03014, doi:10.1029/2008GC002332.
33<24.2 This section was extruded over millions of years from the slow process of the expansion-contraction cycles at the before mentioned Challenger and Menard fractures zones that lie to the west of the islands at the Pacific Rise divergent boundary. They were offset because the west side of the ridge is dominant in allowable movement. It produces more ridge infill and pushes the ridge axis to the east. As the thermal cycles repeated, the crust to the east was compressed and then fracture in parallel east-west faults, slowly extending the section toward the islands. This, in the simplest mechanical terms, is a jack, progressively moving the faulted section to the east. The Antarctic Plate is subducting under the southern portion of the South American west coast.
33<24.3 Image L. The Mass of the Pacific Plate overwhelmed the smaller Antarctic section, its mechanical advancement easily producing more kinetic energy than the Peru-Chile trench could process into the mantle. You can see the redirected crust compressing into the narrow passage, bending the continental extensions and slowly pushing the island arc out to its present position.
33<24.4 Image L above was acquired from GeoMapApp at http://www.geomapapp.org using Ryan, W. B. F., et al. (2009), Global Multi-Resolution Topography synthesis, Geochem. Geophys. Geosyst., 10, Q03014, doi:10.1029/2008GC002332.
-----------------------------------------------------------------------------------------------------------------
Page 34
Summery
------------------------------------------------------------------------------------------------------------------------------------------------------------------------------------------------------------------
34<24.5 Earth's present-day magnetic field is much stronger than normal. The dipole moment is now 8 × 1022 amps × m2. That is twice the million-year average of 4× 1022 amps × m2. This model's rather fundamental and remarkably simple idea is easily understood with the available knowledge that the Earth's magnetic field generator's energy output does change over million year time periods, and that this slow variability would produce what is seen in the most recent observations at the Mid-Atlantic ridge;
"A 20-Myr record of creation of oceanic lithosphere at a segment of the central Mid-Atlantic-Ridge is exposed along an uplifted sliver of lithosphere. The degree of melting of the mantle that is upwelling below the ridge, estimated from the chemistry of the exposed mantle rocks, as well as crustal thickness inferred from gravity measurements, show oscillations of ,3–4 Myr superimposed on a longer-term steady increase with time. The time lag between oscillations of mantle melting and crustal thickness indicates that the solid mantle is upwelling at an average rate of ,25mmyr, but this appears to vary through time."
34<24.7 This includes the most recent extreme solar magnetic energy level shown at left, correlated in timing and intensity to the documented record of 8.0 Japanese earthquakes seen above and that gives measure to the level of specificity that the model can provide between solar magnetic energy output and specific incidences of plate movement.
34<24.8 The 11,400 year long solar magnetic/climate synchronicity leave little doubt that the mutual inductive coupling of the Sun's solar magnetic generator to the Earth's own magnetic field generator is a driver of the Earth's variable climate history. From the initial warming coming out of the Younger Dryas cold period to the precise correlation of the 8.2 Kiloyear event, and the many other correlations also shown, the solar magnetic connection to climate is well supported in this study.

34<24.16 The evidence shown in this model suggests that the observed increase in ocean warming that has been widely attributed to anthropological atmospheric CO2, is actually due to a strain energy response from the slow and periodic displacement of the mantle which began with a gradual increase in activity just prior to the generally accepted end of the Little Ice Age around 1850. These sporadic pulses of thermal content moved gradually from the crust/mantle boundary into the deep ocean where it was then transported through PDO, ENSO and AMOC circulations to the surface where it then warmed the atmosphere.
Please contact me and share your thoughts,
Marc Linquist - Researcher - at;